Boeing 737-700
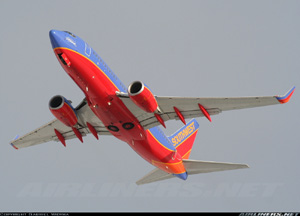
from Chicago Midway International Airport
Photo copyright Gabriel Widyna - used with permission
Southwest Airlines Flight 1248, N471WN
Chicago, Illinois
December 8, 2005
On December 8, 2005, Southwest Airlines Flight 1248 overran the runway during landing at Chicago Midway International Airport. The airplane rolled through a blast fence, an airport perimeter fence, and onto an adjacent roadway where it collided with an automobile before coming to a stop. A passenger in the automobile was killed, one other occupant received serious injuries, and three others in the automobile received minor injuries. Of the 103 passengers and crew aboard the airplane, eighteen passengers received minor injuries, and the airplane was substantially damaged.
The National Transportation Safety Board (NTSB) determined that the probable cause of the accident was the pilots' failure to use available reverse thrust in a timely manner to safely slow or stop the airplane after landing, which resulted in a runway overrun. This failure occurred because the pilots' first experience and lack of familiarity with the airplane's autobrake system distracted them from using reverse thrust during the challenging landing.
Listed contributing factors were Southwest Airlines' 1) failure to provide its pilots with clear and consistent guidance and training regarding company policies related to arrival landing distance calculations; 2) programming and design of its onboard performance computer which did not present inherent assumptions in the program critical to pilot decision making; 3) plan to implement new autobrake procedures without a familiarization period; and 4) failure to include a margin of safety in the arrival assessment to account for operational uncertainties.
Also contributing to the accident, as stated by the NTSB, was the pilots' failure to divert to another airport with more favorable landing conditions and the absence (at Midway Airport) of an engineering materials arresting system (EMAS), which was needed because of the limited runway safety area beyond the departure end of the runway.
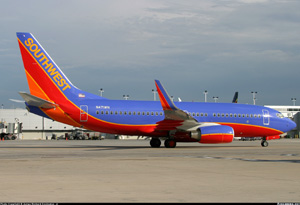
in August 2004
Photo copyright James Richard Covington - used with permission
History of Flight
The accident occurred on the first leg of a scheduled three-day trip that originated in Baltimore. The departure from Baltimore was delayed approximately two hours due to deteriorating weather conditions in the Chicago area. The captain was the pilot flying, and the first officer was the monitoring pilot for this leg. Once en route, the flight crew received weather updates for Midway Airport indicating that runway 31C would be the active runway (changed from 04R), and that braking action reports were mixed, reporting good or fair braking action for the first half of the runway and poor braking action for the last half. Incidental to this flight was the first planned operational use of the automatic braking system, per a new Southwest Airlines policy. Neither pilot had previously used the autobrake system.
Approximately 25 minutes prior to landing, while in a holding pattern, the first officer entered revised weather, runway conditions, and wind information into the Onboard Performance Computer (OPC) in order to determine the required landing distance on runway 31C. The reported wind conditions (090° at 11 knots) resulted in a tailwind component of eight knots. Southwest Airlines is limited to landing with less than a ten knot tailwind, but per company policy flight operations manuals limit tailwinds to less than five knots if runway braking action is reported as poor. Post-accident statements and cockpit voice recorder tapes verified that the pilots were aware of the limitation and believed that if braking action reports were poor for the entire runway, they would not be able to land.
The first officer entered a number of scenarios into the OPC using both fair and poor braking action reports separately, as the OPC would not accommodate mixed reports. Based on his inputs, with fair braking action for the entire length of the runway, the airplane would come to a full stop 560 feet short of the departure end of the runway. Using poor braking action for the entire length yielded a stopping point 40 feet short of the departure end. Further discussion between the pilots also resulted in a decision to divert to an alternate airport if the tailwind component increased to above ten knots or if braking action reports indicated poor braking action for the entire runway length.
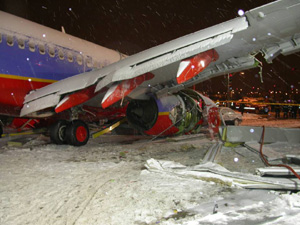
- NTSB Docket Photo
Upon receiving a landing clearance, the first officer was informed that the wind had decreased to nine knots (from the previous 11), and braking action was reported as good for the first half of the runway and poor for the second half. The airplane touched down in the designated touchdown zone at a speed of 124 knots. The ground spoilers deployed and, within one second of touchdown, the automatic braking system began to operate.
Following touchdown the captain stated that he attempted to deploy the thrust reversers but had difficulty moving the levers to the reverse thrust position. He further stated that he felt the anti-skid system begin cycling after touchdown, but that the cycling stopped and the airplane seemed to accelerate. He stated that he then applied manual braking (which disconnects the automatic brakes), but did not continue with his attempt to deploy the thrust reversers. He later stated to investigators that he believed the use of the autobrakes distracted him from the thrust reversers after his initial attempt to deploy them.
The first officer also stated that he felt a reduction in the airplane's deceleration, and also began using manual braking. He subsequently saw that the thrust reverser levers were still in the stowed position, moved the captain's hand away from the levers, and initiated deployment of the reversers about 15 seconds after touchdown. The engines reached full reverse thrust about 18 seconds after touchdown, and approximately 500 feet from the end of the runway. An animation, adapted from an NTSB animation of the accident sequence, is available below:
The airplane ran off the departure end of the runway, through the runway safety area (RSA), a blast fence, and a navigational aid antenna. The airplane then crossed an airport road, went through an airport perimeter fence, and onto a public roadway. Upon entering the public roadway, the airplane struck an automobile before coming to rest near an intersection at the northwest corner of the airport.
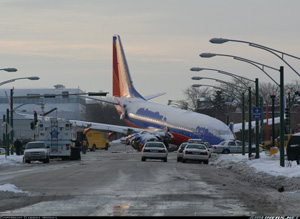
Photo copyright Gabriel Widyna - used with permission
737 Braking System
On the 737, each of the four wheels on the main landing gear has a multi-disc hydraulically powered brake. The brake pedals in the flight deck provide independent control of the left and right brakes. The brake system includes: the normal brake system, an alternate brake system, a brake accumulator, antiskid protection, the autobrake system, and the parking brake. The nose wheels are not equipped with brakes. The alternate brake system functions automatically if the hydraulic pressure for the normal system is low or fails. If hydraulic pressure for both the normal and alternate brake systems is lost, trapped hydraulic pressure in the brake accumulator can still provide several brake applications or a parking brake application.
Antiskid protection is provided in the normal and alternate brake systems. The normal brake system provides individual antiskid protection to each main gear wheel. When the system detects a skid, the associated antiskid valve reduces brake pressure on the affected wheel until the skidding stops. The alternate brake system provides similar protection except that protection is provided to main wheel pairs rather than individual wheels.
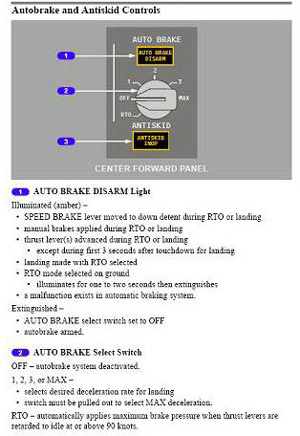
Excerpted from Boeing Operations Manual
The automatic braking system, when armed, automatically applies airplane brakes after touchdown or during a rejected takeoff. Autobrakes were used initially after landing in this accident; however, the pilots applied manual braking (disarming the automatic brakes) relatively early after touchdown. The autobrake system provides four increasing levels of airplane deceleration, and settings are identified as OFF, 1, 2, 3, and MAX, with a separate setting identified as RTO. For landing, the system is armed by moving an instrument panel mounted switch to the desired deceleration setting. The system is generally armed prior to landing, but will begin functioning after landing if autobrakes are selected before the airplane decelerates through 60 knots. Following touchdown, the brakes are automatically applied after both throttles are retarded to idle and the main wheels spin up.
To maintain the selected deceleration rate, autobrake pressure is reduced as other devices, such as thrust reversers, and spoilers are employed and contribute to the overall deceleration. The deceleration level can be changed during autobraking by rotating the selector to a higher or lower setting. The system brings the airplane to a complete stop unless disconnected by the pilot. During landing on a dry runway, the MAX setting results in a deceleration rate that is less than that from full pedal (full hydraulic pressure) braking. On a wet runway, MAX will generally result in application of maximum hydraulic pressure in order to achieve the desired deceleration rate, which may be the same as that produced by maximum pedal braking. In general, a higher setting provides greater deceleration. However, on a wet or slippery runway, maximum deceleration capability may be achieved at one of the lower settings, and deceleration may not be improved by selecting a higher setting.
The RTO function is used only for takeoff and provides automatic braking in the event that a takeoff needs to be discontinued. With the RTO function armed and wheel speeds greater than 90 knots, moving the throttles to the idle position will initiate RTO automatic braking. The RTO setting uses maximum braking, i.e., maximum system hydraulic pressure, and will bring the airplane to a complete stop unless disconnected by the pilot.
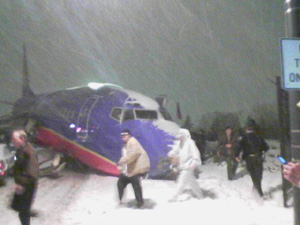
At the time of the accident, Southwest Airlines had planned to implement a policy of requiring the use of autobrakes under certain landing conditions. The policy had been intended to be in effect beginning on December 12, four days after the accident. Previous policies did not allow the use of autobrakes as the Southwest Airlines fleet had not been fully equipped with autobrakes. Southwest Airlines had provided self-study training modules on the autobrake system and related company procedures, which both pilots on the accident flight had completed. Southwest Airlines crews were also provided with a series of bulletins that repeatedly delayed the implementation start date, the most recent of which delayed the date from an earlier date to December 12. The most recent bulletin, indicating the delay in implementation to December 12, was issued on the day of the accident. Both pilots indicated to the NTSB that they had failed to notice the delayed implementation notification. CVR evidence and post-accident statements indicated that both pilots believed the autobrake policy was in effect, when in fact it was not. A previous notification to crews indicated that the autobrake policy would be in effect as soon as materials were available in the cockpit. On the day of the accident, "flow" cards and checklists with autobrake information had been placed in Southwest Airlines airplanes.
Speed Brake/Ground Spoiler System
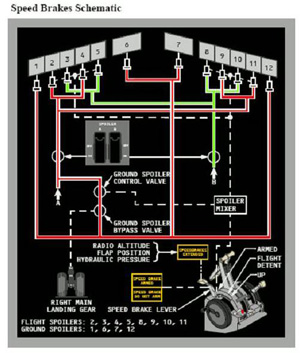
Excerpted from Boeing 737 Operations Manual
View Larger
The speed brakes/spoilers consist of 12 hydraulically powered panels on the upper surface of the wing that function as both in-flight speed brakes and on-ground deceleration and lift spoiling devices. Two panels on each wing are identified as ground spoilers and are powered by a separate hydraulic system from the other four panels on each wing. When the speed brake lever is moved in flight, the flight spoilers are extended symmetrically. When the speed brake lever is moved on the ground, all 12 panels are extended. The flight spoilers can also serve as roll controls, and will function asymmetrically to assist the ailerons in rolling the airplane when cockpit wheel deflections (roll demand) reach threshold deflection levels.
For landing, the speed brake system is armed while still airborne, and following touchdown, once the throttles have been retarded to the idle position and main gear wheels have spun up to 60 knots, the eight flight spoilers deploy. Compression of the right main landing gear strut opens the ground spoiler shutoff valve, deploying the four ground spoilers. If wheel spin up is not detected, transition of the air/ground logic system from air mode to ground mode (resulting from compression of either main landing gear strut) causes the speed brake lever to move from the armed position to the ground deploy position. If the speed brakes were not armed prior to touchdown, actuation of the thrust reversers additionally results in deployment of all 12 spoiler panels. Spoilers can also be deployed manually by moving the spoiler handle to the deployed position. Aft motion of the spoiler handle increases spoiler deflection. If the main throttles are advanced out of the idle position during landing roll, the spoilers will retract.
The NTSB determined that the spoilers functioned normally during this accident.
Thrust Reversers
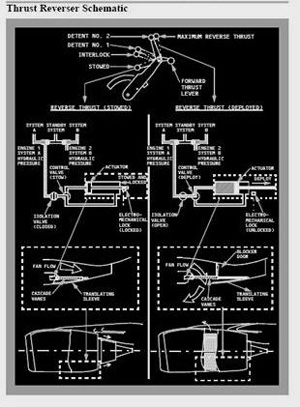
Excerpted from Boeing 737 Operations Manual
Each engine on the 737-700 is equipped with hydraulically operated thrust reversers consisting of left and right translating sleeves. When commanded on landing, or during an RTO, the reverser sleeves move aft, causing blocker doors to open and deflect fan discharge air forward to produce reverse thrust. The thrust reverser is designed and intended for ground use only. If normal hydraulic power is not available for either or both reversers, hydraulic power is available through the standby hydraulic system.
The reverser system arms when the radio altimeter system senses an altitude of less than ten feet, or when the air/ground safety system transitions to ground mode. Movement of the reverse thrust levers is restricted until the forward thrust levers are in the idle position. Once the system is armed and the airplane is on the ground, aft movement of the reverse thrust levers actuates the reverser sleeves. Once the sleeves have fully translated, further movement of the reverse thrust levers increases the level of reverse thrust. Throttle interlocks prevent movement of the reverser levers until the forward levers are in the idle position, and further, prevent an increase in reverse thrust until the reverser sleeves are fully deployed. Forward motion of the reverser levers decreases the reverse thrust level, and moving the levers fully forward to the stow position will result in stowage of the reverser sleeves and cancellation of reverse thrust.
In flight, an automatic restow system compares the actual reverser position and the commanded position. In the event of incomplete stowage or uncommanded movement of a reverser sleeve, the auto-restow circuit functions to automatically drive the reverser sleeve to the stowed position.
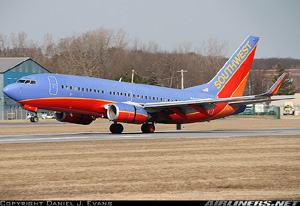
Showing Deployed Thrust Reverser
Photo copyright Daniel J. Evans - used with permission
On the accident flight, reverse thrust was not selected until approximately 15 seconds after touchdown. The captain had stated that he attempted to deploy the reversers but was having difficulty. Following touchdown he further stated that he noticed a large amount of anti-skid system activity and sensed that the airplane had stopped decelerating. This prompted him to cease his attempts to deploy the reversers and to begin maximum effort manual braking. Fifteen seconds after touchdown, the first officer noticed that the reversers had not been deployed, and deployed the reversers himself. The reversers were fully deployed approximately 18 seconds after touchdown and approximately 500 feet from the departure end of the runway. The NTSB ultimately cited the lack of timely thrust reverse application as a primary cause of the accident. Braking conditions were sufficiently poor that the lack of reverse thrust effectively eliminated any safety margins that may have existed and allowed the airplane to depart the end of the runway, resulting in the accident.
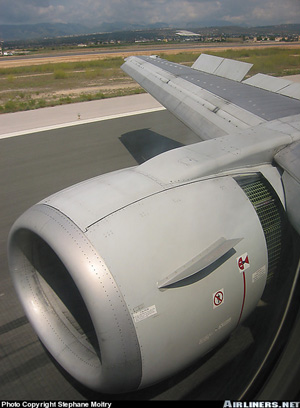
and Extended Ground Spoilers
Photo copyright Stephane Moitry - used with permission
Airplane Stopping Performance
n most operational landing scenarios, the brakes, spoilers, and thrust reversers are employed in combination to bring the airplane to a stop. Reverse thrust is generally most effective at high speeds, and becomes less effective as the airplane slows down. Normal operating procedures also require that reverse thrust be cancelled prior to the airplane coming to a stop. On the 737, reverser cancellation speed is 60 knots. The cancellation speed is based on engine operating considerations and avoidance of adverse engine operating characteristics.
Ground spoilers are also most effective at higher speeds and upon initial deployment in addition to providing drag, also "spoil" wing lift, transferring the airplane weight to the landing gear and making initial braking more effective. The anti-skid system can function more efficiently when airplane weight is supported by the landing gear.
In most landing situations, the brakes are the primary deceleration device. On a dry runway, while reverse thrust and ground spoilers aid in decelerating the airplane, they are not necessary to bring the airplane to a stop. As runway conditions become more slippery due to water, snow, ice, etc., the brakes become less effective, and the airplane can become more dependent on reverse thrust and ground spoilers for deceleration. If automatic braking is used, at lower settings such as 1 or 2, reverse thrust and spoilers may provide the initial deceleration rate and allow the onset of braking to occur later in the landing roll. At higher settings, automatic braking will be initiated almost immediately after touchdown, but upon achieving the target deceleration rate for the selected setting, brake pressure will be reduced, providing a side benefit of an incremental reduction in brake wear. In situations where the target deceleration rate is not achievable, such as an extremely slippery runway, the combination of brakes (automatic or manual), thrust reverse, and ground spoilers provides the maximum deceleration capability.
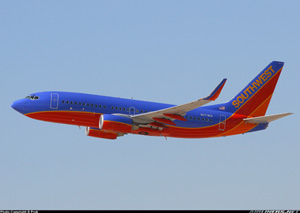
Photo copyright Niels Schieck - used with permission
Dispatch Criteria and Landing Distance Factors
14 CFR 25.125 requires the determination of landing distances on dry, smooth, hard-surfaced runways. The landing distance is defined as the horizontal distance over the ground from an altitude of 50 feet to full stop and is required to be included in the airplane performance section of the approved flight manual. Landing distances are determined during certification flight testing as a demonstration of the maximum performance capability of the airplane and are generally not considered representative of landing distances in airline service. As a result, 14 CFR 121.195 requires the addition of safety factors, defined as a percentage of landing distance. These factors, applied at airplane dispatch (prior to takeoff) and based on the expected landing weight and approach speed, effectively require that the landing runway be longer than will actually be necessary to land and come to a complete stop if the maximum performance capability of the airplane were exercised.
If the landing runway is expected to be wet, an additional factor is applied at dispatch to account for an expected degradation in braking capability and additional required stopping distance. Neither the 14 CFR part 25, nor 14 CFR part 121 requirements for determination of landing distances allow consideration of the effects of reverse thrust. Therefore, if reverse thrust is used on landing, the actual landing distances can be significantly shorter than those determined prior to dispatch. Determination of pre-dispatch landing distances, when destination field lengths may be limited, can be a factor in determination of allowable takeoff weights and/or passenger loading. Landing field length requirements (including the distance factors) will define the allowable landing weight. The landing weight plus the weight of anticipated enroute fuel consumption can determine the allowable dispatch takeoff weight, such that arrival landing weight is within the requirements for the landing runway.
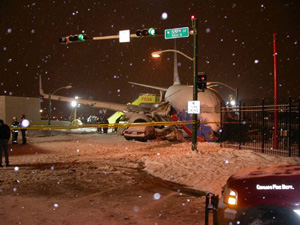
Once the airplane is dispatched, operational regulations do not specify what must be done to assess landing performance on arrival. The only explicit requirement is that landing operations must be suspended or restricted when conditions are hazardous. Operating requirements do not specify whether the effects of reverse thrust can be considered in an after-dispatch landing performance assessment, or whether safety factors (safety margins) should be applied to the calculated landing distance. As a result, different operators follow different practices regarding landing distance assessments after dispatch. In an FAA survey conducted after the accident, it was determined that half of the operators surveyed did not have policies in place to assess landing distances at the time of arrival, even when conditions affecting the landing distance had deteriorated from those existing at the time of dispatch. For those operators performing a landing distance assessment, not all operators applied a safety margin, while some applied the same factors that were applied at dispatch. Some included the effect of reverse thrust, while others did not.
Depending on the specific procedures and allowances practiced by an operator, a disparity can result between the anticipated landing distance determined at dispatch and the actual landing distance determined at the time of arrival. While the dispatch landing criteria included distance factors, real-time calculations, based on the actual airplane weight at the time of arrival, could result in actual landing distances that require a higher percentage of the available runway length than did the dispatch landing calculations. Further, if the arrival runway conditions are different than those anticipated at dispatch, such as the runway being more slippery due to inclement weather, there is no requirement to apply safety factors, such as an additional distance margin. For many carriers, if the available runway is longer than the calculated landing distance, the landing is permitted.
An animation of the various aspects of landing distance determination describes the factors considered and relates the dispatch criteria to operational landing distance assessments. A portion of this animation is included in other modules in this library; however, information specific to this accident has been added to clarify some details of this accident. (View Landing Performance Animation below.)
For airplane performance related calculations, Southwest Airlines uses an onboard performance computer (OPC) to aid flight crews in determining acceptable airplane performance and aid in decision making regarding the acceptability of making a takeoff or landing in real, rather than anticipated, conditions. The OPC can perform a number of calculations, including weight and balance, takeoff performance, en route performance, and expected landing distance performance and stopping margins. The OPC contains airport information (runway length available), and will accept airplane weight and configuration information, winds, and runway surface conditions (braking action reports) in calculating actual takeoff or landing distances. The OPC will provide an alert to the flight crew if the airplane cannot stop on the available runway and will display a negative stopping margin. Southwest Airlines policies did not allow landings in tailwinds of more than 10 knots on runways where braking action was reported as good or fair. Landing tailwinds were limited to five knots if braking action was reported as poor.
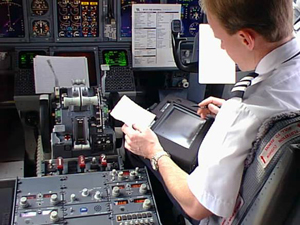
Photo copyright Southwest Airlines - used with permission
If the computed tailwind component exceeds these values, the OPC displays the stopping margin associated with the limit value (five knots) rather than the actual value. In the case of the accident flight, the resulting tailwind component was eight knots, and was used in calculations when braking action reports of better than poor were entered in the OPC. The NTSB accident report stated that when the calculation was repeated using poor braking action, the eight-knot component was displayed, but calculations were based on the five-knot limit. This resulted in a displayed stopping distance that was incrementally better than the actual airplane performance would have been, and included very slight positive margins, indicating that the landing would require all but approximately 50 feet of the available runway. This calculation also included the performance increment provided by the thrust reversers, although during the accident, reverser actuation was delayed, resulting in a negative impact on stopping performance, as stated by the NTSB in the accident report.
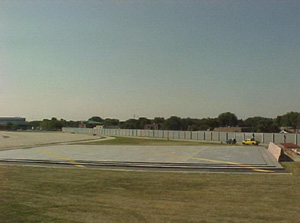
of an Airport - NTSB docket Photo
Runway Safety Areas
A runway safety area is defined by the FAA as, "the surface surrounding the runway prepared or suitable for reducing the risk of damage to airplanes in the event of an undershoot, overshoot, or excursion from the runway." Following a Southwest Airlines runway overrun accident at Burbank, California in 2003, the NTSB issued recommendations regarding runway safety areas. The NTSB recommended that all airports certificated to 14 CFR part 139 upgrade runway safety areas, wherever feasible, to meet the minimum standards established in FAA Advisory Circular 150/5300-13. Further, the NTSB recommended that the same airports install engineered materials arresting systems (EMAS) where the RSAs could not be made to meet the minimum standards in 150/5300-13. The FAA agreed with these recommendations and stated that FAA Order 5200.8 established a program to bring RSAs up to current standards wherever possible.
According to the advisory circular, Midway airport should have been equipped with RSAs that extended 1,000 feet beyond the end of each runway and that were 500 feet wide. At the time of the accident, runway 31C, on which the accident occurred, included an RSA that extended 82 feet beyond the end of the runway and was 500 feet wide. An FAA study of this RSA, conducted in 2000, stated that it did not appear to be practical to achieve the RSA standards for this runway, as the runway could not be realigned on site, and extension of the RSA for the current runway alignment would require relocation of several city streets and acquisition of many businesses and homes in the area. The FAA did, however, instruct the Chicago Department of Aviation to "explore all options to bring the RSAs into full conformance with FAA standards.
In 2003 the Chicago Department of Aviation stated in a letter to the FAA that there were no alternatives for achieving a standard RSA at Midway Airport. The letter stated that the runway could not be shortened and still meet operational requirements for aircraft. Additionally, extension of the RSA would require acquisition of, and major impacts to, surrounding businesses and neighborhoods, public roadways, and public utility infrastructure. Finally, the installation of an EMAS bed was assessed, but insufficient space existed without shortening the runway.
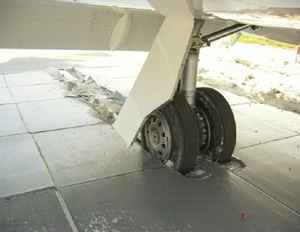
After the accident, the Chicago Department of Aviation sent a letter to the FAA with regard to installing a non-standard EMAS bed on runway 31C. The NTSB also asked the EMAS manufacturer to model the accident scenario and estimate the effect of a non-standard EMAS installation. The manufacturer estimated that an EMAS bed 229 feet long would fit in the overrun area of runway 31C, and that, based on the airplane's exit speed of 53 knots, a 737 landing under the conditions of the accident airplane would stop on an EMAS bed after travelling 206 feet. The simulation estimated that the accident airplane could have been travelling as fast as 58 knots when exiting the runway and still stop on a 229-foot EMAS bed. The NTSB indicated the study predicted that an EMAS installation would have stopped the accident airplane before it departed airport property.
In 2006 the FAA approved the installation of non-standard EMAS beds at Midway Airport, and by early 2006 the first 170 feet of an EMAS bed had been installed, with an additional 40 feet planned.
Runway Snow Removal and Surface Condition Reports
At the time of the accident, Midway Airport had snow removal procedures in place. According to the airport snow removal manual, snow removal and anti-icing are conducted from runway end to runway end. Removal operations are coordinated through the control tower, which is intended to minimize disruption of operations. An operations supervisor in the control tower also receives pilot braking action and surface condition reports and relays these reports to snow removal personnel in order to optimize snow removal operations. Runway friction testing is conducted following removal operations in order to provide information to aircraft regarding the current state of the runway surface.
On the day of the accident, snow removal operations had been ongoing. Snow removal involves the use of runway brooms, snowplows, a snow blower, deice machines, and runway friction testing equipment. Runway 31C had been cleared five times in the five- and one-half hours prior to the accident, the most recent clearing having occurred approximately 30 minutes prior to the accident. Runway friction measurements were in the general range of values that are typically associated with "good" braking conditions. The runway surface condition report was updated approximately 25 minutes prior to landing of the accident aircraft, though snow continued to fall during the period between the condition report and the accident.
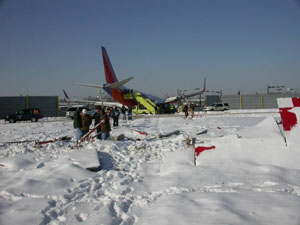
Three methods are typically used to characterize runway surface conditions: observations of runway contaminant depth and type; vehicle friction measurements; and pilot braking action reports. Numerous studies have been conducted over several decades that have attempted to correlate various types of surface condition reports with actual stopping performance. To date, while these studies continue, the FAA has stated that a reliable correlation between various surface condition description methods and actual braking performance has not been established. Pilot braking reports are subjective and can vary depending on the type of airplane and deceleration methods employed. Further, some disparity can develop due to time delays between observations and actual operational input following an airline stop.
Other authorities, most notably Transport Canada and the European Aviation Safety Agency (EASA), do provide correlations of stopping capability between runway surface conditions. Transport Canada provides a correlation, known as the Canadian Runway Friction Index (CRFI), which relates friction values obtained from decelerometers to airplane stopping capability on ice, snow, or loose snow/substrates. The CRFIs are based on contaminant depth and provide a value ranging from 0 to 1, with braking performance increasing as the CRFI increases.
Beginning in January 1996, a number of international organizations, including the United States, formed the Joint Runway Friction Measurement Program intending to define an International Runway Friction Index (IRFI), similar to the CRFI. At the time of this accident, the IRFI had not been correlated with aircraft, and no guidance relative to airplane landing performance has been published.
The NTSB made 23 findings relative to this accident, discussing crew qualifications, use of reverse thrust, use of automatic brakes, landing conditions, landing surface condition guidance, and EMAS. The complete text of the recommendations, including the probable cause, is available here: NTSB Findings
A link to the official NTSB report for this accident is available here: NTSB Report
The NTSB issued eight recommendations and one urgent recommendation as a result of this accident. The eight recommendations addressed arrival landing distance assessments, onboard electronic computing devices, Southwest Airlines' guidance on runway surface conditions, and thrust reverser procedures. The urgent recommendation addressed application of reverse thrust credit in landing performance calculations. The complete text of the recommendations is available here: NTSB Recommendations
As a result of previously conducted studies into runway overrun factors, the NTSB issued a series of recommendations ranging from the need for airport snow removal plans to the formation of industry groups to develop standardized criteria for pilot braking action reports, and evaluations of runway friction measuring devices.
Additionally, following a 1999 accident, the NTSB issued a recommendation to require the use of automatic brakes on wet or slippery runways or in high crosswind conditions.
Finally, as a result of a Southwest Airlines runway overrun in 2003, the NTSB issued two recommendations regarding runway safety areas (RSA). The complete text of these related recommendations is provided here: Related Recommendations
14 CFR 25.125 Landing
This regulation provides the means to determine, during airplane certification, the landing distances that will be provided in the FAA approved flight manual.
14 CFR 121.195 Airplanes: Turbine engine powered: Landing limitations: Destination airports
This regulation specifies the dispatch-related destination landing requirements that must be met prior to takeoff from the airport of origin. This regulation specifies the landing distance factors for dry and wet runways and establishes the requirement for an alternate landing airport.
Further, in 1990, the FAA issued Advisory Circular 121.195(d)-1A which provides a means to determine operational wet runway landing distances, based on established flight manual landing distances and the distance factors required by 14 CFR 121.195. This advisory circular superseded an earlier version that contained essentially the same information.
Additionally, in 1978, the FAA issued Advisory Circular 91-6A which provides information, guidelines, and recommendations concerning the operation of turbojet aircraft when water, slush, and snow are on the runway.
14 CFR 121.533 Responsibility for operational control: Domestic operations.
This regulation places the responsibility for the safe operation of the flight jointly with the operator, pilot in command, and dispatcher as appropriate to the type of operation being conducted.
14 CFR 121.601 Aircraft dispatcher information to pilot in command: Domestic and flag operations.
This regulation requires dispatchers to keep pilots informed of conditions, such as airport and meteorological conditions, that may affect the safety of the flight.
14 CFR 121.603 Facilities and services: Supplemental operations.
This regulation requires pilots to stay informed of conditions, such as airport and meteorological conditions, that may affect the safety of the flight.
Dispatch planning landing distance calculations are required by FAA and international aviation authorities. However, FAA regulations do not require or standardize arrival landing distance assessments, and do not specify safety margins for such assessments. Flight 1248 was dispatched with appropriate landing distance factors based on the assumed landing weight, and on projected time of arrival runway conditions. Upon arrival however, the pilots were allowed to use actual distances calculated by the OPC that included performance credit for thrust reversers and did not include the operational factors applied at dispatch. This effectively allowed consideration of the entire available runway surface for calculation of the landing distance, negating the distance factors that had been applied at dispatch.
Certification landing distances are established as demonstrations of a maximum performance capability of the airplane. Operational landing distance requirements add factors to the actual distances as dispatch safety margins. This effectively results in a requirement that landing runways be longer than will actually be necessary. Application of operational factors also provides a predictive method to determine operational suitability for landing at a specific airport but does not address the actual conditions at the time of landing. As a result, operational rules allow flight crews to determine actual landing distances, based on onboard calculations appropriate for actual airport conditions, and airplane weight and configuration at the time of arrival. The use of one set of information to determine factored landing distances for airplane dispatch and another set of information to determine actual landing distance at time of arrival, eliminated any safety factors upon which dispatch had been based, and allowed landing on a runway that was too short for the prevailing conditions.
Relative to runway surface conditions, the industry has not yet developed a consistent method for determination or reporting surface conditions and runway slipperiness. Three methods are currently used: type/depth of contamination, pilot braking action reports, and friction measurements from ground friction measuring devices. However, there are no standardized definitions of terms used to describe types of runway contaminant or pilot braking action, pilot braking action reports are subjective and dependent on the type of airplane, operational procedures, and amount of braking used and where on the runway that braking is employed. A reliable correlation between friction measurement device readings and airplanes has not been developed. Runways must be closed in order to run a friction measurement device down the runway, and the devices cannot be used in many conditions where friction information is critical. There are also issues with the timeliness of taking readings with the friction measuring devices, and with matching reported runway surface conditions (however they are reported) with the airplane performance data available to the operator.
- Delayed actuation of reverse thrust following touchdown
- Lack of runway safety areas equipped with engineered arresting materials systems to provide additional deceleration in the event of a runway overrun
- Determination of real-time landing distance that did not include distance or safety margins
Tower Air
In December 1995 a Boeing 747 operated by Tower Air departed the runway during an attempted rejected takeoff at John F. Kennedy International Airport. As a result of this accident, the NTSB recommended the adoption of a program to establish runway friction measurements that are "operationally meaningful to pilots and air carriers for their slippery runway operations...and minimum coefficient of friction levels for specific airplane types below which airplane operations will be suspended."
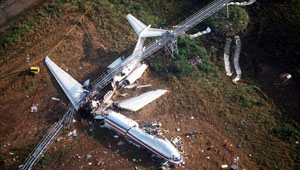
The FAA has participated in an ongoing program to establish a means to reliably determine runway friction values and provide a uniformly interpreted system of braking action reports that would provide air carrier crews with information necessary for landing distance assessments and determination of landing suitability.
American Airlines
On June 1, 1999, American Airlines Flight 1420, a McDonnell Douglas DC-9-82 (MD-82), N215AA, crashed after it overran the end of runway 4R during landing at Little Rock National Airport in Little Rock, Arkansas. After departing the end of the runway, the airplane struck several tubes extending outward from the left edge of the instrument landing system localizer array located 411 feet beyond the end of the runway; passed through a chain link security fence and over a rock embankment to a flood plain located approximately 15 feet below the runway elevation; and collided with the structure supporting the runway 22L approach lighting system. As a result of their investigation, the NTSB recommended that automatic brakes be used for landings during wet, slippery, or high crosswind conditions.
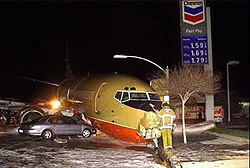
See accident module
Southwest Airlines
In May 2003, a 737 operated by Southwest Airlines overran the runway after landing at Burbank, California. The airplane departed the end of the runway, ran through a blast fence, crossed a road and came to rest in a gas station. Following this accident, the NTSB recommended the installation of engineered materials arresting systems on all runways that were not able to meet the standards established in FAA advisory circular 150/5300-13, "Airport Design."
Landing Distance Assessments
On June 7, 2006, the FAA published an "Announcement of Policy for Landing Performance After Departure for All Turbojet Operators." The announcement stated that the FAA considered a 15-percent margin as the minimum acceptable safety margin between the expected actual airplane landing distance and the available runway landing distance at the time of arrival. The FAA planned to issue mandatory Operations Specification N8400.C082 to all 14 CFR Part 91 subpart K, 121, 125 and 135 turbojet operators. The proposed operations specification would have required the following:
- Use of an operationally representative air distance.
- Use of data at least as conservative as the manufacturer's data.
- Use of the worst reported braking action for the runway during landing distance assessments.
- Operator's addition of an extra margin of at least 15 percent to the landing distance performance calculations, and
- Require Principal Operations Inspectors to ensure that operators' flight crew and dispatcher training programs provide information about all aspects and assumptions of actual landing distance performance determinations.
The FAA had intended that operators comply with the operations specification by October 2006. However, considerable industry opposition was encountered, and the FAA decided not to issue the mandatory operations specification, but to pursue formal rulemaking. In the interim, the FAA issued Safety Alert for Operations (SAFO) 06012 containing the same information as the proposed operations specification. Compliance with the SAFO was voluntary. The SAFO suggested that operators:
- Establish procedures that require assessing the landing distance as close as practicable to the time of arrival if conditions have adversely changed since the preflight landing distance assessments.
- Apply a safety margin of 15 percent to the calculated landing distance when such assessments are required at the time of arrival; and
- Provide information regarding all aspects of actual landing distance performance assessments in their flight crew and dispatcher training programs.
On December 6, 2007, the FAA established a Takeoff and Landing Performance Assessment (TALPA) Aviation Rulemaking Committee (ARC) to review regulations affecting certification and operation of airplanes and airports for airplane takeoff and landing operations on runways contaminated by snow, slush, ice, or standing water and provide advice and recommendations to:
- Establish airplane certification and operational requirements (including training for takeoff and landing operations on contaminated runways.
- Establish landing distance assessment requirements, including minimum landing distance safety margins, to be performed at the time of arrival.
- Establish standards for runway surface condition reporting and minimum surface conditions for continued operations.
The TALPA ARC delivered their recommendations for landing operations on April 15, 2009, and for takeoff operations on July 7, 2009. The FAA is currently conducting trial evaluations of some of the recommendations with certain airports and operators. The FAA expects to pursue formal rulemaking based on the TALPA ARC recommendations and the trial evaluations.
Runway Safety Areas (RSAs)
Following the May 2003 Southwest Airlines runway overrun in Burbank, California, the NTSB issued recommendations addressing runway safety areas. In response, the FAA issued Order 5200.8, "Runway Safety Areas Program," establishing a program to bring all RSAs up to current standards whenever possible. During the NTSB public hearings relative to Flight 1248, the FAA stated that while not all RSAs being upgraded would meet the dimensional standards specified in the order, RSA upgrades were ongoing, including installation of an engineered materials arresting system in the available areas at Midway Airport.
Southwest Airlines
Following the accident, Southwest Airlines revised its operational policies and procedures to correct shortcomings identified by the NTSB. Revision included:
- Modifications to sections of their flight crew operations manual to reinforce company policy requiring use of the most restrictive braking action report in the OPC when making landing distance assessments, and provided additional training specific to braking action reports, including mixed conditions.
- Updates to the OPC to standardize the use of thrust reverser credit in landing distance assessments and modified the output screens of the OPC to indicate the amount of reverse thrust necessary to obtain the calculated landing distances.
- Reinforcing thrust reverser policies to emphasize the need for immediate deployment of reverse thrust after landing.
- Updates to procedures for the use of reverse thrust to be consistent with Boeing guidance.
- Clarifying guidance regarding the responsibility of the monitoring pilot to monitor reverser deployment.
- Implementing an autobrake familiarization period before autobrake use and revised the flight crew operations manual to include a technical description of the system. Southwest Airlines required that pilots complete at least four landings on dry runways with ample stopping margin before using the autobrake system on a routine basis.
- Adding a 15-percent safety margin to arrival landing distance calculations and revising the OPC to include the additional margin.
Though regulatory actions were undertaken by the FAA in several different areas, no airworthiness directives were issued as a result of this accident.
Airplane Life Cycle:
- Operational
Accident Threat Categories:
- Inclement Weather / Icing
- Incorrect Piloting Technique
- Landing / Takeoff Excursions
Groupings:
- Approach and Landing
- Automation
Accident Common Themes:
- Organizational Lapses
- Human Error
Organizational Lapses
At the time the 737-700 was certified by the FAA, certification requirements did not allow the consideration of performance improvements provided by use of thrust reversers. Landing distances provided in the FAA-approved airplane flight manual therefore did not include the effects of reverse thrust. Further, in consideration of the requirements of operational regulations, FAA-approved landing distance information included runway distance factors for both dry and wet runways that were required to be applied at the time of dispatch. Applying these factors at dispatch could affect the allowable takeoff weight, based on the expected arrival weight and runway length available for landing. If the projected arrival landing weight resulted in a factored landing distance that exceeded the available runway length, the dispatch takeoff weight would have to be reduced to accommodate the landing field length requirements.
However, per Southwest Airlines policies, at the actual time of arrival, landing distance calculations were allowed to include the effects of reverse thrust, and actual landing distances were allowed to require the entire available runway length. For the accident, the difference between dispatch and actual arrival landing distance calculations, based on arrival airplane weight/configuration and meteorological conditions, resulted in an actual landing distance calculation that did, in fact, require the use of the entire runway. No additional margins were available. The delay in application of reverse thrust resulted in an unintended increase in the landing distance and resulted in the runway overrun. If additional landing distance factors had been required at the time of arrival, it would have become apparent that the available distance was inadequate, and the flight would have diverted to an airport with a longer runway, or with acceptable runway conditions.
Also, Southwest Airlines implemented a new policy to use automatic brakes under certain landing conditions without including a familiarization phase as part of the implementation program. As a result, even though the program had been delayed, the accident flight crew was under the impression that the program was to begin on the day of the accident. The flight crew had no prior experience with the autobrake system and did not know what to expect in terms of braking performance on a slippery runway or the system characteristics. Though the system operated properly, landing on a slippery runway resulted in a flight crew perception that the airplane was not decelerating when autobrakes began operating, so the captain concentrated on using manual brakes and did not deploy the thrust reversers, which in this case were critical to the stopping performance of the airplane. Had the autobrake policy included a familiarization period, the flight crew may have been better prepared for the characteristics they experienced and would have deployed the thrust reversers in a timelier manner.
Human Error
Southwest Airlines' company policy did not allow landing in tail winds of greater than 5 knots if runway braking conditions were reported as poor. Southwest Airlines guidance indicated that if runway braking conditions are mixed, then the more critical of the reports should be considered when making the decision to land. For the accident flight, reported runway conditions were a mixture of fair and poor, resulting in a crew decision to land, though tailwinds exceeded five knots and company policies indicated that a landing should not have been attempted. Further, the onboard landing distance computations only used the five-knot company tailwind limit and did not use the actual wind value which exceeded five knots.
The NTSB determined that though airline policy required use of the most critical reported braking conditions, training materials did not adequately address the situation, perhaps contributing to the decision to land. Further, after touchdown, the crew delayed the activation of reverse thrust for 18 seconds. As landing distance calculations included the activation of reverse thrust immediately after touchdown, the delay in activation resulted in an increase in the stopping distance. The airplane was no longer able to stop on the runway.
Following this accident, Southwest Airlines operational documents were revised to require that the most critical braking report be used when mixed reports are provided. Southwest Airlines also implemented pilot training specific to interpretation of braking action reports, including mixed conditions.
The following is not an exhaustive list, but includes a number of accidents similar in circumstances to SWA 1248:
January 2010, American Airlines 737 runway overrun on landing at Kingston, Jamaica
April 2007, Pinnacle Airlines runway overrun on landing at Cherry Capital Airport, Traverse City, Michigan
August 2005, Air France A340 runway overrun on landing at Toronto, Canada
February 2008, Shuttle America ERJ-170 runway overrun on landing in Cleveland, Ohio
May 2003, Southwest Airlines 737 runway overrun on landing at Burbank, California
June 1999, American Airlines MD-80 overrun at Little Rock, Arkansas
See accident module
December 1995, Tower Air 747 runway excursion during an attempted rejected takeoff at John F. Kennedy International Airport in New York
January 1982, World Airways DC-10 runway overrun on landing at Boston, Massachusetts
Technical Related Lessons
Runway stopping performance should consider all factors that can influence stopping capability and include safety margins to mitigate unforeseen or changed conditions. (Threat Category: Landing/Takeoff Excursions)
- At the time of the accident, operational landing distance assessments were not required to include safety margins and were allowed to consider the effects of reverse thrust. Operationally, this resulted in calculation of landing distances that could require the entire available runway length to come to a stop after touchdown. At the time of the accident, the OPC used by Southwest Airlines did not adequately address the wind conditions that existed at the time of the accident, and included the effects of thrust reversers, assuming they were deployed immediately after touchdown. The OPC resulting calculations presented a landing distance that required the entire available runway and did not provide a margin for delayed actuation of deceleration devices. The lack of margins, coupled with the delay in thrust reverser actuation, resulted in the accident.
Common Theme Related Lessons
Introduction of new policies or procedures should be preceded by an evaluation/familiarization period prior to routine usage in operational situations. (Common Theme: Organizational Lapses)
- Prior to this accident, Southwest Airlines had announced a new policy to begin using automatic brakes under certain landing conditions. Though the implementation date had been delayed by Southwest Airlines, the accident flight crew was under the impression that they were to begin using autobrakes on the day of the accident. As a result, when autobrakes began functioning on landing, the captain stated that he was sufficiently distracted by autobrake functioning. His perception was that deceleration was inadequate, and he concentrated on using manual brakes and did not deploy the thrust reversers. Calculated stopping performance included the use of thrust reverse, and little to no stopping margin existed if reversers were not deployed in a timely manner. As a result, the airplane did not stop on the available runway surface, and the accident resulted. Following the accident, Southwest Airlines implemented an autobrake familiarization program requiring each pilot to use the autobrakes on dry runways with adequate stopping margin to acquaint themselves with the autobrake characteristics prior to using them in marginal stopping conditions.