Airbus SAS A320-214
US Airways Flight 1549, N106US
New York City, New York
January 15, 2009
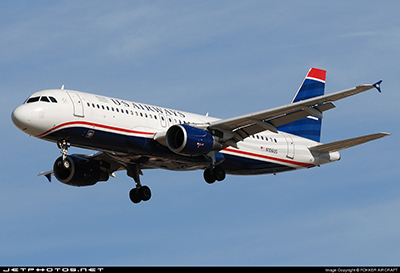
On January 15, 2009, at about 15:25 EST, US Airways Flight 1549, a scheduled domestic passenger flight on an Airbus SAS (Airbus) A320-214, took off from LaGuardia Airport (LGA) in New York City, New York, destined for Charlotte, North Carolina. On board the flight were five crewmembers and 150 passengers, including one lap child. The aircraft was operating on an instrument flight plan, and weather was clear with visibility of ten miles. Less than two minutes after takeoff the aircraft encountered a flock of large birds, which resulted in the ingestion of multiple birds and the near complete loss of thrust in both engines. After an emergency turnback toward LGA and several relight attempts, the pilots ditched the aircraft on the Hudson River about 8.5 miles from LGA. All occupants evacuated the airplane via the forward and over-wing exits and were rescued by nearby responders. One crewmember and four passengers had serious injuries and the aircraft sustained substantial damage.
The NTSB determined the probable cause of this accident was the ingestion of large birds into the two engines, leading to near total loss of thrust in both engines and the consequent ditching of the aircraft on the Hudson River.
History of Flight
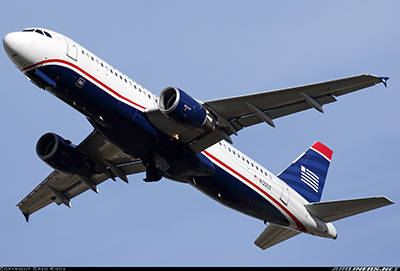
On January 15, 2009, at about 15:25 EST (20:25 UTC), US Airways Flight 1549, an Airbus A320 aircraft powered by two CFM56-5B4/P engines, left New York City’s LaGuardia Airport (LGA) on a scheduled domestic passenger service flight bound for Charlotte Douglas International Airport (CLT), Charlotte, North Carolina, then to Seattle-Tacoma International Airport (SEA) in SeaTac, Washington.
The first officer was the pilot flying and the captain was the pilot monitoring as the aircraft made a routine departure and initial climb. Less than two minutes after takeoff, the captain called out "birds." As the aircraft climbed through 2,750 feet, at approximately 15:27 local time, and approximately four and a half miles from LGA, Flight 1549 encountered a large flock of Canada geese.
Geese entered the fan and core sections of each engine, resulting in near total loss of thrust in both engines. The pilots observed the sound of decreasing engine noise, which was also picked up on the cockpit voice recorder (CVR). The flight data recorder (FDR) showed that after the bird encounter, fan and core speeds of both engines decelerated and airspeed immediately began decaying from 219 knots indicated airspeed (KIAS). The captain called out the rollback and failure of both engines. The captain turned on engine ignition and started the Auxiliary Power Unit (APU), indicated by the red arrow, as shown. He then called, "my aircraft."
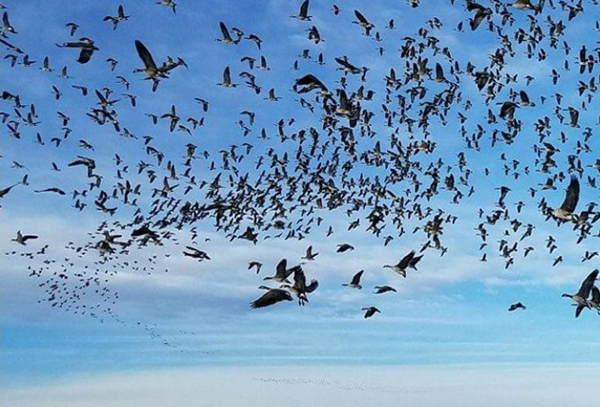
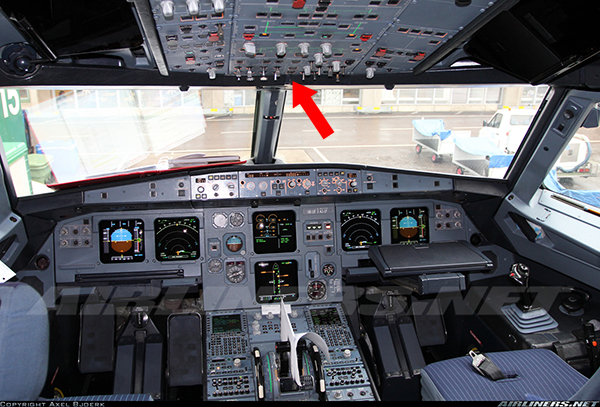
The captain took control of the aircraft as pilot flying and advised the first officer to take out the quick-reference handbook (QRH) and refer to the checklist for loss of thrust in both engines. Altitude peaked at approximately 3,060 feet, and airspeed decreased to approximately 185 KIAS. As altitude decreased, airspeed increased again to approximately 210 KIAS. The first officer, as pilot monitoring, began Part 1 of the three-part, US Airways QRH, ENG DUAL FAILURE checklist. Both pilots realized they did not have the optimum relight airspeed and due to their low altitude could not attain it in a dive. Approximately two minutes after departure, the captain declared an emergency and informed Air Traffic Control (ATC) the aircraft was turning back toward LGA.
ATC provided instructions to turn left on heading 220° for return to LGA and offered LGA Runway 13 for emergency landing. The captain reported they might be unable to reach the airport, telling ATC, "We may end up in the Hudson [River]."
Due to the low altitude and insufficient time before ditching, the pilots only completed the first nine of fifteen steps from Part 1, and none of Parts 2 and 3 of the checklist. Meanwhile, ATC offered LGA Runway 31 for landing, and the captain told ATC "unable." ATC then offered Runway 4, to which the captain replied, "I'm not sure we can make any runway," and asked what was available to his right. ATC confirmed Teterboro Airport (TEB) in New Jersey was to his right.
After the final unsuccessful relight attempt, with the airplane losing altitude and speed, the captain announced to the cabin over the passenger address system, "This is the captain, brace for impact." While the pilots were controlling the airplane and conducting the checklist, they received numerous traffic collision avoidance system (TCAS) and ground proximity warning system (GPWS) alerts. The pilots could not respond to these alerts because of task saturation and the lack of available thrust and therefore chose to ignore them.
ATC offered Runway 1 at TEB for landing, to which the captain said, "We can't do it." When asked by ATC which runway at TEB he would like, the captain responded, "We're gonna be in the Hudson." Between four and five minutes after departure, the aircraft had descended to 200 feet and the airspeed stabilized between 185 and 193 KIAS.
On the captain's command, at around 1530 local time, the first officer extended the flaps to "flaps 2." After flap deployment, as they continued descending, the captain asked the first officer, "got any ideas?" to which the first officer responded, "actually, not." As speed decayed, and amid continued TCAS and GPWS aural alerts, the pilots braced for impact. As the captain pulled back on the sidestick for landing, the aircraft envelope (alpha) protection inhibited the flare.
During final descent, the airspeed reached about 125 KIAS at touchdown. At the same time, angle of attack increased from approximately 8° to approximately 13° at touchdown. Just before 15:31 local time, three and a half minutes after encountering a flock of Canada geese, US Airways Flight 1549 touched down on the Hudson River. Total flight time for Flight 1549 that day was five minutes and eight seconds.
During the ditching, the left engine separated from its wing pylon. The aircraft experienced structural damage to the aft fuselage, resulting in water entering the back of the passenger cabin. The first officer began the evacuation checklist. The captain opened the flight deck door, announced to the cabin an immediate evacuation of the aircraft, and discovered the cabin crew had already begun the evacuation.
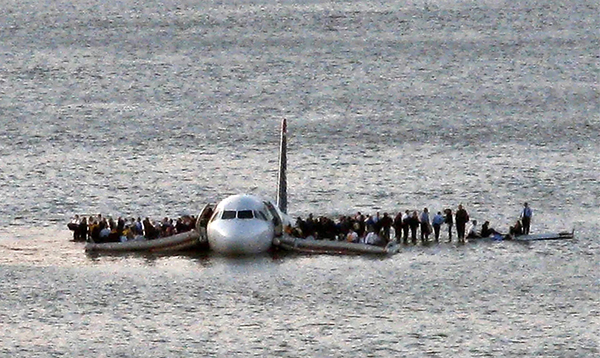
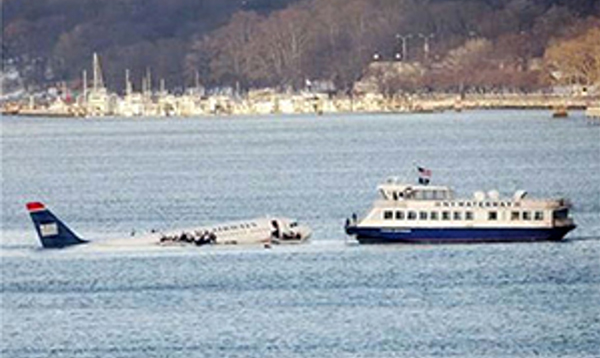
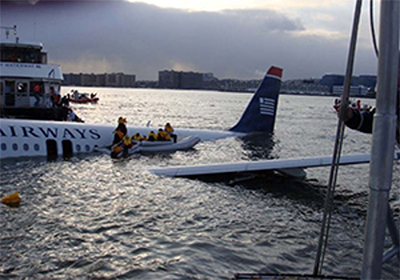
As evacuation efforts began, the door 1 left (1L) escape slide did not properly deploy, resulting in one passenger jumping into the river, where water temperature was approximately 41° F with a wind chill factor of 2° F. The aft exits were unusable due to incoming water, and passengers were instructed to move forward to evacuate. Passengers escaped through the over-wing exits on both sides of the aircraft and stood on the wings awaiting rescue. Passengers immediately began exiting onto the door 1 right (1R) slide and, after the door 1L slide was adjusted, passengers escaped onto both forward slides. The forward slides were then disconnected from the aircraft and used as rafts, per their design. Slides from the over-wing exits were not designed or certified for use as rafts.
New York Waterway Taxis were the first responders to arrive. According to the NTSB final report, the first ferry approached the aircraft within three minutes of the ditching and began rescuing the waiting occupants of Flight 1549, with six more ferries arriving within ten minutes. The New York and New Jersey Offices of Emergency Management, U.S. Coast Guard, and Fire Department of New York also performed water rescue operations.
Within about 26 minutes after take-off from LGA, and within 20 minutes of the ditching, all 150 passengers, including one lap child, and all five crewmembers onboard the aircraft were successfully rescued. They were taken to several nearby locations in New Jersey and New York for medical evaluation and transport. Five people – one cabin crewmember and four passengers – experienced and were treated for severe injuries, while 95 passengers were treated for minor injuries.
Canada Geese
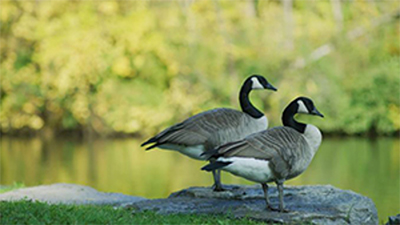
There are two distinct populations of Canada geese in New York, the resident population and the migratory population. Resident geese are identified as such because they spend most of their lives in one area. They live and breed in Southern Canada and the U.S. Migratory geese, on the other hand, form flocks and migrate seasonally, breeding in Northern Canada, then wintering in the U.S.
According to the investigation, Smithsonian National Museum of Natural History, Feather Identification Lab in Washington, DC evaluated numerous samples collected at the accident site from the engines of Flight 1549. The lab determined the accident samples were similar to samples of known migratory geese wintering in the local area at the time and were significantly different from resident populations in the region.
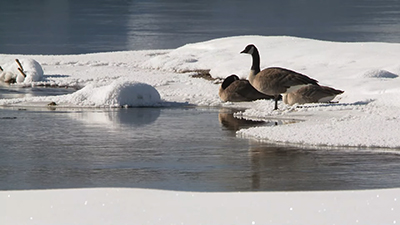
Migrating birds typically return to the same path, called routes or flyways, each year. The flyway used by the migrating Canada geese found in the New York area is the Atlantic flyway, which runs along the eastern coast of North America. According to the encyclopedia Britannica, adult migratory Canada geese range in size from 4.4 to 14.3 pounds, with wing spans as wide as 6.6 feet.
According to the United States Department of Agriculture and FAA Wildlife Strike Report, most reported bird strikes, about 71%, occur below 500 feet above ground. January, when this accident occurred, is the second least likely month for bird strikes.
Dual-engine power losses from bird ingestion are rare. Add to this, Flight 1549 encountered the flock of migratory geese, at around 2,800 feet, and in the month of January, all of which contributed to making this accident a very low probability event.
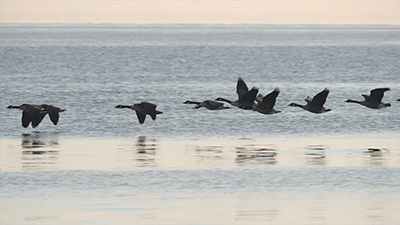
Currently, the Port Authority of New York/New Jersey takes a multi-tiered approach to wildlife mitigation. This includes habitat modification, wildlife dispersal, daily wildlife patrols, bird monitoring, laughing gull nest surveys, mammal spotlight surveys, insect control, water management, egg-oiling, and, when necessary, trapping and shooting. The Port Authority has a staff wildlife biologist who assists the wildlife supervisors. The wildlife supervisors provide around-the-clock airport coverage. The location of the bird strike for this accident was outside the mitigation area for any of the local airports or government authorities.
Engines
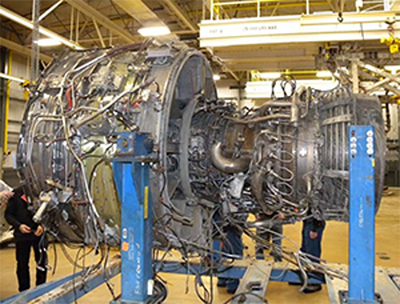
During this event, the size and number of birds that entered each engine – at least two geese weighing approximately eight pounds each – significantly exceeded the airworthiness standard. After the strike, the left engine rolled back to around idle, and the right to sub-idle. A single engine at idle could not provide enough thrust to maintain altitude and sustain the flight.
The Flight 1549 aircraft was powered by two CFM56-5B4/P engines. The engines were compliant with airworthiness standards in effect at the time of certification. This engine model was required to withstand bird ingestion for medium and small flocking birds and still produce adequate power to sustain flight. The certification test requirement for large birds at that time was for a single, four-pound bird aimed at the critical area, to be ingested into one engine. While the rule does not require that an engine continue providing thrust, the engine must be designed so that the ingestion of a large bird does not result in release of hazardous fragments through the engine case – referred to as uncontained engine failure. The engines were tested and compliant with these requirements.
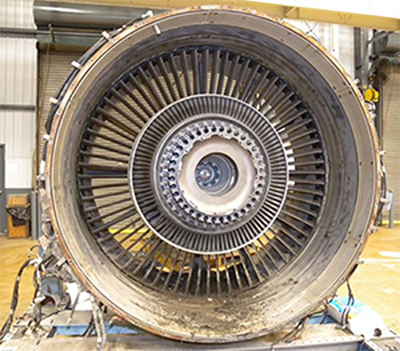
The standard for large bird testing has since increased to one four-, six-, or eight-pound bird, depending on engine size, into one engine. The current large bird requirement for an engine of this size is still one four-pound bird ingested into one engine. Because of their size, these engines were (and still are) exempt from large flocking bird testing.
The investigation determined that at least two geese, weighing approximately eight pounds each, entered the cores of each of the two engines. The core is where the engine derives most of its power. When birds enter the fan or bypass portion of the engine, they typically continue through the bypass duct and exit out the aft end of the engine. Birds entering the engine core are more likely to cause internal engine damage that could result in engine stall and loss of thrust. The accident investigators for Flight 1549 identified many distorted, damaged, and fractured engine blades, vanes, and numerous other components. Both engines had significant mechanical damage to the high-pressure compressor flow path, with multiple broken blades.
In addition to foreign object ingestion requirements, the CFM56-5B4/P engines were subject to blade containment requirements. An engine must contain damage from failure of critical components, in accordance with the applicable requirements, to prevent engine parts from breeching the engine case and striking critical surfaces of the aircraft. Both engines successfully contained the blades in this accident.
Pilot Training
Pilots for any commercial airline are required to complete various types of academic and operational training. All pilots receive training specific to each aircraft "type" they are qualified to fly (e.g., Airbus A320) to obtain a "type rating." Pilots may hold multiple type ratings.
When working toward a new type rating, pilots first complete initial training. This training is extensive and takes weeks or months to complete to gain the appropriate systems knowledge and skill needed to safely fly the aircraft. While the first officer of Flight 1549 was a seasoned pilot, he had recently completed initial type rating training on the A320. Recurrent training is designated-interval training required to maintain skills. The frequency of recurrent training varies across companies, training content, and aircraft models, and may be modified if design changes are made to the aircraft.
Crew Resource Management (CRM) is one type of required pilot training and focuses on situation awareness, communication skills, teamwork, task allocation, and decision making. In summary, CRM teaches the flight crew how to work together in the safest and most efficient way. Some of the training is company or operator specific; for example, how the flight crews share and accomplish duties in the flight deck and divide roles and tasks between the pilot flying and the pilot monitoring.
Both initial and recurrent training consist of classroom instruction, computer-based training, and motion-based simulator training, and includes a final evaluation called a check ride. The purpose of the simulator training is to provide the pilot with a close approximation of the real-world environment. Different weather conditions can be replicated, and pilots can virtually fly to or from almost any airport. Pilots are exposed to numerous standard and non-standard procedures, as well as a variety of failure and emergency scenarios. The syllabus and content of pilot training is generally defined by the operator and approved by the FAA or a responsible foreign authority, according to their applicable regulations.
The investigators noted that Airbus did not provide training to pilots, at the time, about the effects of alpha protection features on the airplane’s response to pilot sidestick pitch inputs. Such training provides pilots with a better understanding of the aircraft response upon entering alpha protection mode. Investigators also raised concerns that pilots did not receive, nor were required to receive, low-altitude, dual-engine failure training that would challenge their critical thinking and exercise their skills in task shedding, decision-making, and proper workload management.
Pilots demonstrate and practice dual-engine failure scenarios in the simulator during initial training. Since the longest phase of most flights is the cruise (high altitude) phase, the US Airways dual-engine failure training was limited to failures occurring at high altitude (e.g., 20,000 feet). At US Airways, the training exercise typically concluded when one engine was restarted, with drift down to approximately 8,000-feet altitude. Training was geared toward teaching engine relight methods and was not intended as preparation for an off-airport, loss-of-thrust landing. No training was conducted, nor required, for low-altitude, dual-engine failures. The only engine-failure training conducted and required at low altitudes was for single-engine failure.
Ditching training was conducted only during initial training, and only in ground school because it could not be replicated in the simulator. The captain observed that landing on the water was difficult, compared to a typical hard surface landing, due to "a much more uniform visual field, less contrast, and fewer landmarks." This lack of contrast and visual references, while not common, may give pilots the perception of being higher above the water's surface than they are.
During an emergency, the primary job of the pilot flying is to maintain control of the aircraft. The pilot monitoring evaluates the alerts and determines the appropriate action. There were no actions the pilots on this flight could have taken during the emergency, to prevent the descent. Despite the shortcomings of the training that was provided, the flight crew of Flight 1549 worked together well, relying on all their training, skills, and extensive experience to safely ditch the aircraft, which was essential to the survivability of this accident.
Checklists
Emergency and abnormal procedure checklists are critical to pilots, as they include reminders of items that may be trained but are not often encountered. While pilot and crew training are extensive, there are some rarely experienced failure conditions that may not appear in the training curriculum or may be forgotten over time. This is a primary reason checklists and QRH design are critical in the event of an emergency.
The A320 has an electronic centralized aircraft monitor (ECAM) system, which presents data to the pilots on the engine/warning and system display. When the flight warning computer (FWC) detects an aircraft system failure, the failure type and flight crew actions to be taken (i.e. procedures or checklists) are displayed on the ECAM. Some abnormal events are not detected by aircraft systems. For such events the pilots were to use the US Airways QRH, which contained emergency and abnormal procedures.
The back cover of the US Airways QRH used on this flight contained a list of procedures, with associated page numbers, called "ECAM Exceptions," for six particular high-criticality events, among them Engine Dual Failure. Since the first officer of Flight 1549 had recently completed US Airways initial type rating training on the A320, he immediately recognized the failure of both engines as an ECAM exception, quickly reviewed the back cover of the QRH, and located the ENG DUAL FAILURE checklist. While the first officer retrieved the checklist, the captain turned on engine ignition and engaged the APU. The pilots did not reach the step in the checklist where the APU was called for; however, the captain made the choice to turn it on early in the emergency, knowing the air provided by the APU would allow a starter-assisted air-start, if possible, and provide electrical power and maintain additional systems.
The complete US Airways checklist can be found here: ENG DUAL FAILURE Checklist
Task saturation during an emergency may lead a flight crew to become fixated on one item (i.e., tunnel vision), or to lose their place in a checklist. This is especially true if pilots must alternate between checklists or are distracted by other alerts or duties. To minimize the need for pilots to alternate between checklists, sometimes multiple checklists are combined into one, which can lead to lengthy and complex procedures. Checklist brevity allows a pilot to ensure all steps are covered in a rapidly developing, high-pressure environment, as occurred with this accident. The checklist used during this event lacked such brevity.
The ENG DUAL FAILURE checklist that US Airways procedures required pilots to use was long and complicated and not designed for a low-altitude emergency. Similar to the training for such a failure, the checklist was developed for a high-altitude event, where with loss of both engines a flight crew would normally have ample time to complete a lengthy checklist. Due to the low altitude of the airplane, and checklist length and complexity, the Flight 1549 crew was unable to complete most of the checklist. There was no checklist available for a low-altitude engine dual failure, and no way to quickly assess the ability of engines to restart. Due to the extent of damage to both engines, there was no action the pilots could have taken to recover thrust and arrest or reverse the descent.
The ENG DUAL FAILURE checklist used in this accident was made up of three parts:
- Part 1, procedures for differentiating between a no fuel remaining and a fuel remaining condition and attempting an engine restart
- Part 2, procedures to follow depending on whether an engine restart is successful or unsuccessful
- Part 3, procedures in the event a forced landing or ditching is required
The checklist used had numerous steps and decision points, and the flight crew completed only nine of the fifteen steps in Part 1. They did not reach Part 2 procedures, which were based on whether an engine restart was successful or unsuccessful, and they did not reach Part 3, which included the ditching portion of the checklist. Instead, because there was no time or altitude left, the pilots made the best decisions they could, based on skill, experience, and memory.
Checklist Part 1, procedures to attempt relight of the engines, involve setting the engines to idle and accelerating (i.e., diving) to an optimum relight speed of 300 KIAS. Diving to increase speed was not an option, due to the aircraft’s low altitude. The pilots made several unsuccessful engine relight attempts, to no avail. The captain attempted to keep the aircraft at green dot speed, which is the best glide speed when engine restart is impossible.
Step d., early in Part 1 of the checklist, directed the crew to determine a landing strategy. Realizing the engines were not restarting and believing that they did not have the speed nor altitude to safely reach an airport runway, the captain decided to ditch the aircraft on the Hudson. Investigators determined that this decision provided the best chance for survivability of this accident.
While the pilots were controlling the aircraft and conducting steps in Part 1 of the checklist, they received numerous TCAS and GPWS alerts and ATC communications. The crew could not respond to these alerts because of task saturation and lack of available thrust, and therefore chose to ignore them. Investigators found that there were 15 GPWS alerts triggered during the descent, from 300 feet to impact, and that no low-speed (i.e., low-energy) aural alert was triggered during that time. In an emergency, when pilots are saturated with visual information, they may not see primary flight display indications of low airspeed. Auditory warnings are designed to provide this awareness. Due to Airbus alert prioritization hierarchy, low-speed warnings were inhibited by the GPWS alerts; so, pilots did not receive the auditory low-speed warnings. Maintaining sufficiently high airspeed increases likelihood of maintaining sufficient energy to reduce descent rate during the flare to land.
Investigators determined the guidance provided in the ditching portion of the ENG DUAL FAILURE checklist was not consistent with a separate, stand-alone ditching checklist (which the pilots also did not use). The ENG DUAL FAILURE checklist, at the time, included procedures for preparing and configuring the aircraft for ditching but lacked a step to select the GPWS system and terrain alerts to OFF, a step that was included in the stand-alone ditching checklist. While the flight crew did not reach the ditching portion of the ENG DUAL FAILURE checklist, investigators recommended that certain procedures for ditching should be consistent in all applicable checklists.
The stand-alone US Airways QRH ditching checklist also assumed one engine was still operating. If Fight 1549 still had one engine operating, ditching would not have been considered and the flight crew would have returned to LGA (or another nearby airport) under power of the one engine. Any checklist or instruction for ditching, with an engine running, did not apply to the dual-engine loss situation this flight crew was facing.
Airplane Performance
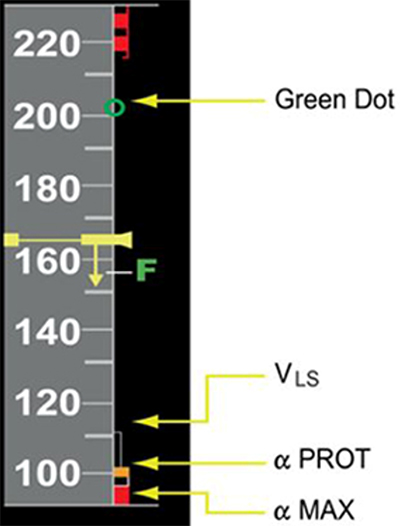
The FAA and other aviation authorities require all large passenger aircraft, like the A320, to be controllable if the aircraft loses all power (as well as other adverse conditions). One of the reasons this flight fared so well after the catastrophic loss of both engines was its ability to perform in such a situation as part of the aircraft design.
Onset of the Emergency
Once an airplane loses all engine thrust, it essentially becomes a glider. Depending on configuration and weight, the aircraft achieves its maximum range and glide capability when flying at the optimum lift over drag ratio. This occurs at "green dot" speed, indicated by a green ring on the airspeed tape, as shown.
For the accident aircraft, given its weight and configuration, this optimum ratio occurred at around 223 KIAS. Flying faster or slower than the optimum glide speed reduces attainable distance. Pilots learn during training about the importance of optimum lift/drag ratio, and green dot speed, to address loss of all engine power when relight is impossible.
To optimize engine restart capability, the ENG DUAL FAILURE checklist directs the pilot to accelerate to 300 KIAS. To achieve this speed, the pilot would need to dive the aircraft, which might be impossible at low altitude. The captain and first officer determined, due to low altitude and airspeed, that reaching optimum relight speed was impossible.
The captain stated during investigations that he was aware of his speed and altitude, which led him to conclude the aircraft would not safely reach any airport. Realizing his best option was to ditch the aircraft, he turned out over the Hudson River and began his approach. As the first officer extended the flaps, the aircraft slowed and both pilots concentrated on the descent, final approach, and ditching.
Airbus Flight Controls
Airbus aircraft have conventional control surfaces consisting of ailerons and spoilers for roll control, rudder for directional control, and elevators for pitch control. They also have trim systems in directional and pitch axes for stabilizing aircraft control. On older aircraft, mechanical connections – cables or hydraulic systems – move the flight-control surfaces. The pilot commands a desired outcome and fine-tunes the input to get the desired response. Generally, the amount of control surface movement is proportional to the pilot’s input commands.
On the A320 and other Airbus aircraft, the rudder uses mechanical control, but the pitch and roll axes use a fly-by-wire (FBW) system, which is electronic control. With the FBW system, there is no mechanical connection from the pilot to the control surface. Instead, the pilot commands a desired outcome, which sends a signal to an electronic flight control system (EFCS). The computer calculates the control surface command to achieve the desired response. The EFCS then commands the control surface by electronic signal to the actuator that moves the surface. This FBW design allows the implementation of several envelope protection functions for safety. These protections include bank angle limiting, acceleration (G) limiting, and angle of attack (AOA) limiting (which relates to aircraft pitch). As part of envelope protection, when a pilot attempts a command that exceeds one of these limits, the EFCS intervenes and does not execute the command. For Flight 1549, as the captain pulled back on the sidestick to slow and flare the aircraft for landing on the river the Airbus A320 envelope protection limited aircraft AOA. This effect was unknown to the pilots at the time and Airbus training did not include the effects of alpha protection features on the airplane's response to pilot sidestick pitch inputs under these circumstances.
The flight controls have three operational modes based on the health of all the computer, peripheral, and hydraulic-generation systems. They include Normal Law, Alternate Law, and Direct Law. During the entire flight, the accident aircraft stayed in Normal Law, due to engine rotation output and APU electrical power supply to the required electrical buses. Normal Law on the Airbus refers to a "pitch rate/G command" control law. This means that, at normal speeds the pilot can control the pitch rate, or G. If the pilot lets go of the side-stick controller (i.e., no command input is given) the airplane has neutral stability, meaning there are no forces on the controller to adjust the trim.
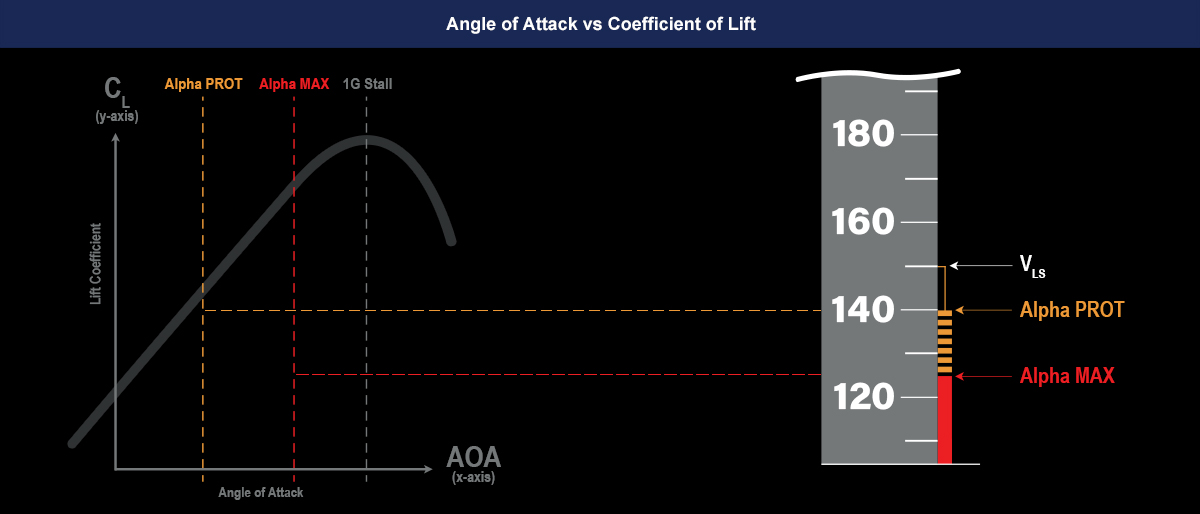
Airbus high AOA protection is available from takeoff to landing and allows pilots to achieve and maintain the optimum possible performance, while mitigating the risk of stall or loss of control. This system displays alpha control speeds to the pilot as black and amber or red bands on the airspeed tape. These speeds relate to AOA and stall protection, as shown. A pilot flies a normal approach at VLS, the lowest selectable speed, approximately 1.23 times stall speed. VLS provides sufficient safety margin above stall. As the airplane slows further, approaching stall, it reaches VAlpha PROT, which is the first alpha protection point, determined by aircraft weight and configuration.
In alpha protection mode, the system switches elevator control from normal mode to alpha protection mode. As the aircraft slows further, while staying above alpha max speed, pilot input to the sidestick results in command by the EFCS, producing nose-down trim to increase stability. If the pilot continues to pull aft on the sidestick, the airplane reaches the alpha max protection limit. This is the alpha max speed corresponding to the top of the red strip on the airspeed tape. It is the maximum AOA available in Normal Law, which prevents stalling the aircraft. If the pilot releases the sidestick, the aircraft returns to alpha protection AOA.
Final Approach
As the aircraft descended to the river, the crew did not have time to complete the ENG DUAL FAILURE checklist. The GPWS and TCAS inhibit switches were not selected off, creating distracting GPWS and TCAS alerts and annunciations which overrode low-speed warnings.
As Flight 1549 continued its descent, the captain attempted to hold VLS as the first officer configured the flaps. As the aircraft decelerated below VLS, the high AOA protection activated and stayed activated until the aircraft impacted the water.
Ditching and Structural Failure
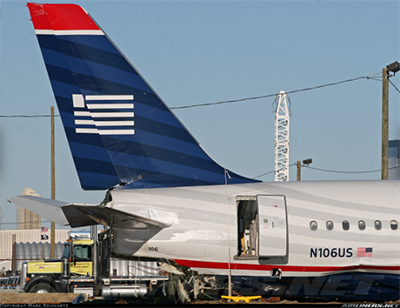
Structural – Investigation
Commercial passenger aircraft designs are required to meet stringent safety requirements, including for the unlikely event of a ditching. US Airways Flight 1549 sink rate, at 13 feet per second, far exceeded the rate assumed for certification of the aircraft, which was 3.5 feet per second. This higher rate led to external pressures acting on the fuselage that investigators estimated to be more than twice the certification values.
NTSB investigators concluded that aircraft damage sustained in the ditching was caused by the high-energy impact at the aft fuselage, followed by post-impact movement of the airplane through the water.
Despite the severity of the impact, the design of the aircraft fuselage structure protected the aircraft occupants.
- The fuselage did not break up on impact or during post-impact motion in the water. This helped minimize immediate injury to occupants, caused by the airplane, and made it possible for them to escape.
- The flotation time of the airplane allowed occupants to leave the airplane and assemble on the wings or enter slide/life rafts.
- The cabin remained intact, and the floor and furnishings retained sufficient integrity to allow all occupants to evacuate the aircraft safely.
- The airplane exits maintained sufficient integrity to allow all occupants to evacuate the aircraft safely.
Despite the high vertical impact velocity and resulting damage to the aircraft, all occupants were protected from critical injury and able to safely evacuate the aircraft.
Conclusion
The NTSB determined the probable cause of the US Airways Flight 1549 accident was "the ingestion of large birds into the two engines, resulting in near total loss of thrust in both engines, and the subsequent ditching on the Hudson River." The events surrounding this accident were influenced by multiple unique factors, including:
Flock of Geese – Overall, this bird strike was exceptionally unlikely. Canada geese that encountered Flight 1549 were migratory birds, wintering in the area (not migrating). According to the United States Department of Agriculture and FAA Wildlife Strike Report, most reported bird strikes, about 71%, occur below 500 feet above ground; however, this strike occurred at 2,800 feet. Additionally, this strike occurred in January, which is the second least likely month for a bird strike event in this area.
The magnitude of this bird strike was also unlikely, considering multiple geese of about eight pounds each were ingested by both engines. The flock of geese involved in this accident was exceedingly large and such a phenomenon was unaccounted for in certification requirement at the time the engines were certified, or even to date.
Flight Crew Training and Experience – The pilots of Flight 1549 were recognized by the NSTB for their excellent decision making and crew resource management during the accident sequence, despite a lack of specific training for this scenario.
The pilots were type-rated on the Airbus A320 and had completed all applicable initial and recurrent training. While training took place for a high-altitude, dual-engine loss scenario, no training was available for low-altitude, dual-engine failure. Engine-failure training conducted at low-altitude – at takeoff or near the ground – involved only the loss of a single engine. The NTSB raised concerns about this training deficit, believing low-altitude, dual-engine training for pilots could improve critical thinking and exercise pilot skills in task shedding, decision-making, and proper workload management.
The pilots on Flight 1549 were highly skilled, with both having piloted aircraft for many years. The captain was particularly well-versed on the A320 aircraft's performance capabilities, allowing him to adapt to the emergency. Both pilots, with their professionalism and safety focus, were essential to a positive outcome on this flight.
Checklists and CRM – The checklist available during this accident was designed for a high-altitude, dual-engine failure and was unable to address this low-altitude, dual-engine emergency. The checklist was long and complicated and had to be abandoned long before it could be completed. In addition, prior to starting any checklist instruction, the captain started the APU, knowing it would maintain electrical power and other systems on the aircraft.
The flight crew's skills allowed them to focus on their situation, maintain quality communications, and manage tasks, leading to wise decision making and a safe emergency landing. The flight crew ultimately controlled and successfully ditched the aircraft, using their skill, experience, and best judgment, and in an aircraft that was designed to remain controllable and protect its occupants.
Successful Evacuation and Rescue – NTSB investigators recognized the performance of the cabin crewmembers (i.e., flight attendants) in taking immediate action to safely expedite the evacuation of the airplane, which was instrumental to the safe outcome for aircraft occupants.
Investigators also credited the immediate and appropriate response of the ferry boat operators and other emergency responders, and their fortunate proximity to the accident site, as significant to the successful outcome of the rescue.
Emergency Equipment – The NTSB noted the good fortune that the aircraft was equipped for water emergencies. Although not required for this flight, the aircraft was outfitted with extended over-water operations equipment, such as the slide rafts used by many during the evacuation and rescue.
Acknowledgement
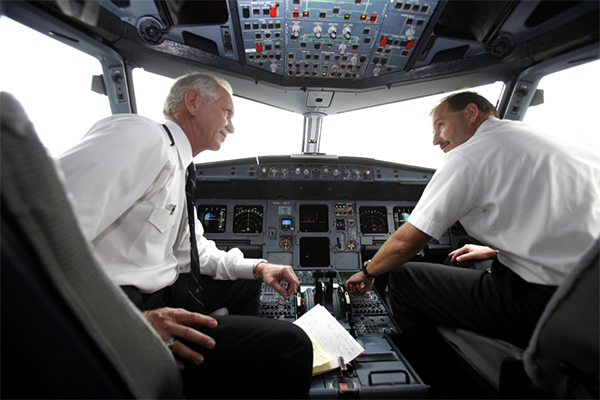
The FAA appreciates Chesley Burnett "Sully" Sullenberger III, who was captain on the January 15, 2009 US Airways Flight 1549, for his generosity and expert assistance in the preparation and review of this "Lessons Learned from Civil Aviation Accidents" module.
Captain Sullenberger has been dedicated to the pursuit of aviation safety his entire adult life. He served as the U.S. Ambassador and Representative to the International Civil Aviation Organization (ICAO) in 2022. He is a keynote speaker to audiences around the world and he continues his advocacy for safety in global air travel.
After learning to fly at age 16, Captain Sullenberger attended the United States Air Force Academy. In addition to a bachelor's degree in psychology, he also has two master's degrees, one in industrial psychology from Purdue University and one in public administration from the University of Northern Colorado. He also has an honorary Doctorate of Letters from Purdue University.
Sullenberger began his flying career as a fighter pilot with the United States Air Force, where he advanced to achieve the rank of captain. Following his service in the Air Force, in February 1980, he became an airline pilot with Pacific Southwest Airlines, later acquired by US Airways, until his retirement from commercial flying in March 2010. He still flies privately, having logged more than 20,000 lifetime hours of flight time.
In addition to his continued work in aviation safety advocacy, Sullenberger is the author of two books: Highest Duty: My Search for What Really Matters and Making a Difference: Stories of Vision and Courage from America's Leaders.
Thank you, Ambassador, Captain Sullenberger.
The NTSB determined the probable cause of the accident was the ingestion of large birds into the two engines, resulting in near total loss of thrust in both engines, and the consequent ditching of the aircraft on the Hudson River.
NTSB findings: View all
NTSB accident report: US Airways Flight 1549
As a result of this accident, the NTSB issued 34 new safety recommendations and one previously issued (reiterated) safety recommendation. Of those new recommendations, 25 were issued to the Federal Aviation Administration, one was issued to the United States Department of Agriculture, and eight safety recommendations were issued to the European Aviation Safety Agency (EASA).
NTSB recommendations: View all
From the NTSB report: The A320-100/200 series airplanes were type certificated for operation in the United States by the FAA under a bilateral airworthiness agreement between the United States and French governments. The FAA approved the A320 type certificate (TC) on December 15, 1988. The A320-214 model was approved on December 12, 1996.
Prior to September 28, 2003, CFM engines, including the CFM56-5B4/P, were certified under a bilateral agreement between the French Direction Generale de L'Aviation Civile (DGAC) in compliance with the Joint Aviation Authorities (JAA) Joint Aviation Regulations - Engines (JAR-E) requirements and the FAA under Title 14, Code of Federal Regulations (14 CFR) Part 33. Since 2003, the engines are certified under EASA (as aviation oversight authority of European member states) and FAA standards.
Some technical regulations of the two authorities were not harmonized (they had differing certification requirements). As such, Airbus and CFM were required to use the more stringent regulation from each agency's requirements to ensure compliance to both standards.
These regulations, relevant to this accident, are shown in the order of the accident sequence, with the exception of operations.
Engines, Bird (Foreign Object) Ingestion and Blade Containment
In this accident both the size and number of birds were greater than the standards, to which the engines were tested and certified. The CFM56-5B engine family passed all its bird ingestion testing for certification. According to the NTSB report, the CFM56-5B passed additional testing, required by the French authority, for medium and large birds during certification testing conducted in 1992, 1993, and 1996. The imposed requirements for some tests exceeded the applicable FAA standard, § 33.77, at the time. The required weight of medium flocking birds for these engines was 2 1⁄2 lbs (rather than 1 1⁄2 lbs), the time to maintain no more than 25-percent loss of thrust was 20 minutes (rather than 5 min), and the number of successful tests required was five (rather than one).
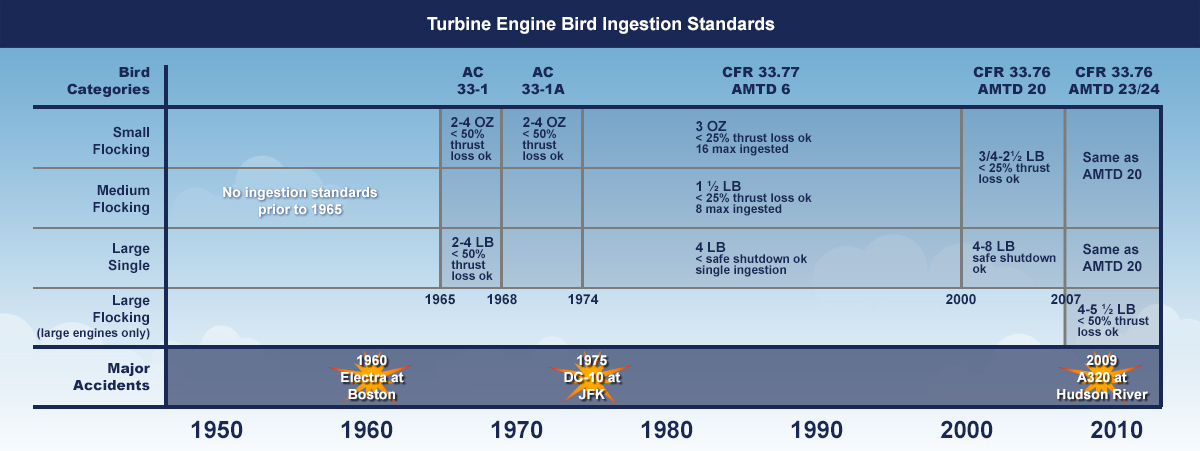
The engines were able to contain the high-pressure compressor blades and other components upon failure.
§ 33.94 Blade containment and rotor unbalance tests, Amdt. 33-10
(a) Except as provided in paragraph (b) of this section, it must be demonstrated by engine tests that the engine is capable of containing damage without catching fire and without failure of its mounting attachments when operated for at least 15 seconds, unless the resulting engine damage induces a self-shutdown, after each of the following events:
(1) Failure of the most critical compressor or fan blade while operating at maximum permissible r.p.m. The blade failure must occur at the outermost retention groove or, for integrally bladed rotor discs, at least 80 percent of the blade must fail...
Aircraft Controllability
The pilots were able to control the aircraft (turning, steering, descending, landing) from the time of impact with the geese until reaching their chosen landing location on the Hudson River, as required by 14 CFR § 25.671.
§ 25.671 General, Amdt. 25-23
(d) The airplane must be designed so that it is controllable if all engines fail. Compliance with this requirement may be shown by analysis where that method has been shown to be reliable.
Structure
The aircraft structure protected the occupants and performed as intended. Items of mass within the cabin remained properly restrained, preventing further hazard to occupants.
14 CFR § 25.561 General, Amdt. 25.23
(a) The airplane, although it may be damaged in emergency landing conditions on land or water, must be designed as prescribed in this section to protect each occupant under those conditions.
[(b) The structure must be designed to give each occupant every reasonable chance of escaping serious injury in a minor crash landing when--
(1) Proper use is made of seats, belts, and all other safety design provisions;
(2) The wheels are retracted (where applicable); and
(3) The occupant experiences the following ultimate inertia forces [acting separately] relative to the surrounding structure:
(i) Upward--2.0g.
(ii) Forward--9.0g.
(iii) Sideward--1.5g.
(iv) Downward--4.5g, or any lesser force that will not be exceeded when the airplane absorbs the landing loads resulting from impact with an ultimate descent velocity of five f.p.s. at design landing weight.
(c) The supporting structure must be designed to restrain, under all loads up to those specified in paragraph (b)(3) of this section, each item of mass that could injure an occupant if it came loose in a minor crash landing.
Ditching and Occupant Protections
All 155 occupants on board evacuated safely and without critical injury. Five of the 155 occupants on board sustained serious injuries for which they were treated. Despite structural damage to the aft portion of the aircraft, the aircraft stayed afloat long enough to allow evacuation of all occupants. Doors and windows on this aircraft withstood the pressures generated during the ditching and performed as intended.
14 CFR § 25.801 Ditching, Original
(b) "Each practicable design measure, compatible with the general characteristics of the airplane, must be taken to minimize the probability that in an emergency landing on water, the behavior of the airplane would cause immediate injury to the occupants or would make it impossible for them to escape"
(d) "It must be shown that, under reasonably probable water conditions, the flotation time and trim of the airplane will allow the occupants to leave the airplane and enter in the life rafts required by 14 CFR § 25.1415. If compliance with this provision is shown by buoyancy and trim computations, appropriate allowance must be made for probable structural damage and leakage"
(e) "Unless the effect of the collapse of external doors and windows are accounted for in the investigation of the probable behavior of the airplane...the external doors and windows must be designed to withstand the probable maximum local pressure"
Emergency Evacuation and Rescue
Emergency exits were located forward cabin, mid-cabin, and aft cabin. Even though aft cabin exits were not accessible, all occupants evacuated through the forward and mid-cabin exits.
14 CFR § 25.807 Emergency exits Amdt. 25-55
(e) - Uniformity. Exits must be distributed as uniformly as practical, taking into account passenger seat distribution.
The two forward exit emergency evacuation slides were, by design, also liferafts. Seat cushions and life preserver vests were both available to all occupants; however, only 20% of life vests and 53% of seat cushions were used. Life lines were available at overwing exits, but they were not included in the passenger briefing, not identified in the emergency information card, and not used during the event.
14 CFR § 25.1411 General, Amdt. 25-53
(a) - Accessibility.
(1) Required safety equipment to be used by the crew in an emergency must be readily accessible.
(d) - Liferafts.
(1) The stowage provisions for the liferafts described in § 25.1415 must accommodate enough rafts for the maximum number of occupants for which certification for ditching is requested.
(2) Liferafts must be stowed near exits through which the rafts can be launched during an unplanned ditching.
(3) Rafts automatically or remotely released outside the airplane must be attached to the airplane by means of the static line prescribed in § 25.1415.
(4) The stowage provisions for each portable liferaft must allow rapid detachment and removal of the raft for use at other than the intended exits.
(f) - Life preserver stowage provisions. The stowage provisions for life preservers described in § 25.1415 must accommodate one life preserver for each occupant for which certification for ditching is requested. Each life preserver must be within easy reach of each seated occupant.
(g) - Life line stowage provisions. If certification for ditching under § 25.801 is requested, there must be provisions to store life lines. These provisions must -
(1) Allow one life line to be attached to each side of the fuselage; and
(2) Be arranged to allow the life lines to be used to enable the occupants to stay on the wing after ditching.
All emergency equipment was approved. Due to speed of rescue operations, these features were not critical in this accident. The only onboard life rafts used during the event were the slide-rafts located at the forward doors. The liferafts were equipped with trailing lines, as required.
§ 25.1415 Ditching Equipment Amdt. 25-52
(b) Each liferaft and each life preserver must be approved. In addition -
(1) Unless excess rafts of enough capacity are provided, the buoyancy and seating capacity beyond the rated capacity of the rafts must accommodate all occupants of the airplane in the event of a loss of one raft of the largest rated capacity; and
(2) Each raft must have a trailing line, and must have a static line designed to hold the raft near the airplane but to release it if the airplane becomes totally submerged.
Operating Information
Transport category airplanes must come with operating procedures, as defined in 14 CFR § 25.1585.
§ 25.1585 Operating procedures
(a) Information and instructions regarding the peculiarities of normal operations (including starting and warming the engines, taxiing, operation of wing flaps, landing gear, and the automatic pilot) must be furnished, together with recommended procedures for--
(1) Engine failure (including minimum speeds, trim, operation of the remaining engines, and operation of flaps);
...
(3) Restarting turbine engines in flight (including the effects of altitude);
...
(5) Ditching (including the procedures based on the requirements of § 25.801, 25.807(d), 25.1411, and 25.1415(a) through (e));
Operations
Operators must provide approved and readily useable check procedures, including normal and emergency procedures, to crewmembers, as defined in 14 CFR § 121.315 effective April 01, 1965.
§ 121.315 Cockpit check procedure.
(a) Each certificate holder shall provide an approved cockpit check procedure for each type of aircraft.
(b) The approved procedures must include each item necessary for flight crewmembers to check for safety before starting engines, taking off, or landing, and in engine and systems emergencies. The procedures must be designed so that a flight crewmember will not need to rely upon his memory for items to be checked.
(c) The approved procedures must be readily usable in the cockpit of each aircraft and the flight crew shall follow them when operating the aircraft.
If an airplane is equipped with flotation equipment, operators must brief passengers about the location and use of required emergency flotation means. US Airways Emergency Manual advised flight attendants if the airplane is equipped with both flotation seat cushions and life vests, flight attendants should brief passengers on both types of equipment, including the location and use of life vests. Only flotation cushions were included in the passenger briefing for this flight.
14 CFR § 121.571 Briefing passengers before take-off
(a) Each certificate holder operating a passenger-carrying airplane shall insure that all passengers are orally briefed by the appropriate crewmember as follows:
(1) Before each takeoff, on each of the following:
...
(iv) The location and use of any required emergency flotation means.
...
(b) Each certificate holder must carry on each passenger-carrying airplane, in convenient locations for use of each passenger, printed cards supplementing the oral briefing. Each card must contain information pertinent only to the type and model of airplane used for that flight, including-.
...
(2) Other instructions necessary for use of emergency equipment;...
Crewmember Qualifications, current and historic, are found in 14 CFR, Part 121, Subparts M, N, and O. These rules include training, testing, and qualification requirements for scheduled passenger service crewmembers, and others.
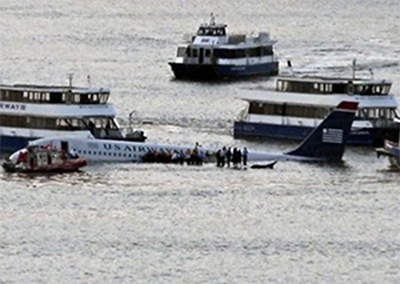
Crew Resource Management – The experience and preparedness of both pilots enhanced their ability to communicate and respond to the emergency as a team. Though they had not met until three days prior to the accident flight, they worked well together, knowing what they each needed to accomplish. Pilot pairing worked to their advantage on this flight.
Two of the factors uncovered during the investigation included lengthy and overly complex checklists, and the absence of a training requirement for low-altitude failure of both engines and unpowered ditching or forced landing. Despite these factors, the flight crew of Flight 1549 was able to manage the emergency and ditch the aircraft with no fatalities.
U.S. Airways adopted the Airbus approach, where the ENG DUAL FAILURE checklist was designed for failures above 20,000 feet and did not consider a dual-engine failure checklist for use at low altitude. A checklist that was overly complex, lengthy, and designed for high altitude was unable to address the issues encountered at low altitude on this flight. Because of this flight crew's strong communication skills and extensive knowledge and ability, they were able to prevent a tragedy.
First Responders – After Flight 1549's successful ditching on the Hudson River, multiple organizations and industries came together to rescue the passengers and crew. These included New York and New Jersey Water Way Ferries, Port Authorities of New York and New Jersey, emergency response and public safety organizations, including local police and fire departments, United States Coast Guard, New York Office of Emergency Management, Red Cross, and ferry terminal operations. The water conditions were near freezing, which could have led to a catastrophic outcome if not for the quick action by these responders, as well as the close proximity of the airplane to these services.
Some of the first responder organizations conducted a water rescue drill just months prior to the accident. All the various organizations have conducted, for example, some combination of routine emergency training, live exercises, drills, table-top exercises, mass-casualty exercises, and were well prepared and able to act quickly during this emergency, leading to the successful outcome.
- Inadequate emergency pilot training for this situation – Training for a low-altitude, dual-engine loss was not provided as it was believed that the chance of encountering this situation was extremely low.
- Overcomplicated and lengthy emergency checklists, inadequate to address this situation – Similar to training, there was no expectation that dual-engine loss at low altitude was a likely scenario. Because of this, there was not a specific emergency checklist available to the pilots for this situation.
- Bird threats – Some bird threats exceed any state of the art of the airplane and its ability to deal with the threat. While the conditions of the Flight 1549 bird strike grossly exceed current standards, the chance of such conditions lining up as they did remains very low.
Dual-engine loss will likely occur at or above 20,000 feet.
- This accident's dual-engine loss occurred at approximately 2,800 feet
- As this was a low-altitude, loss-of-thrust situation, there was not sufficient altitude nor time to recover, had the engines been able
Pilot training and checklists for dual-engine loss at high altitude are sufficient for occurrences at any altitude.
- Flight 1549 dual-engine loss occurred at low altitude
- The pilot training for dual engine loss, only at high altitude, did not provide the knowledge and skills needed to address such an emergency occurring at low altitude
- The checklist, designed for high altitude, was exceedingly lengthy and complex and was impractical for the urgency of a low-altitude, dual-engine loss
Large bird ingestion threats, including flocking, result in failure of only a single engine.
- Large birds (migratory Canada geese) entered both engine cores on Flight 1549, resulting in near complete loss of thrust in both engines
Testing with a single, four-pound large bird is sufficient to represent the threat of large bird encounters in flight.
- The accident flight encountered a large flock of geese, resulting in multiple birds, weighing approximately eight pounds each, entering and damaging both engines
Bird Hazard
Sikorski S-76C++, Petroleum Helicopters, Inc., Amelia, Louisiana, January 4, 2009
A S-76C++ helicopter crashed seven minutes after departure from its home base of Amelia, LA. The helicopter was en route to an offshore oil rig helipad. The helicopter crashed in remote, marshy terrain after experiencing a loss of control event, descending rapidly, and impacting the ground. The pilot, copilot, and six of the seven passengers were killed. Analysis of the audio recordings revealed a loud "bang" which was immediately followed by sounds consistent with "rushing wind." The NTSB determined that the accident was the result of a collision with a red-tailed hawk that fractured the windshield and moved the engine power levers to a low power/idle position.
NTSB accident report: CEN09MA117
Library module: Sikorsky S-76C++
Vickers-Armstrongs Viscount 745D, United Airlines Flight 297, Ellicott City, Maryland, November 23, 1962
November 23, 1962, a Vickers-Armstrongs Viscount 745D airplane struck at least two whistling swans while taking off from Newark, NJ. All thirteen passengers and all four crewmembers were fatally injured. The probable cause of this accident was determined to be the loss of control following separation of the left horizontal stabilizer which had been weakened by a collision with a whistling swan.
NTSB accident report: United Airlines Flight 297
Library module: United Airlines Flight 297
Lockheed Electra, Eastern Airlines, Inc. Flight 375, Boston, Massachusetts, October 4, 1960
On March 10, 1960, a Lockheed Electra turboprop ingested European starlings into multiple engines during takeoff from Boston Logan Airport resulting in a multi-engine power loss. The plane crashed into Boston Harbor, killing 62 people. The accident prompted the FAA to initiate action to develop minimum bird ingestion standards for turbine powered engines.
NTSB accident report: Eastern Airlines Flight 375
Library module: Eastern Airlines Flight 375
Bird Strike Initiatives
-
After the US Airways Flight 1549 accident, a committee was formed under the Aerospace Industries Association (AIA) to review engine bird-ingestion experience in worldwide commercial aviation. The committee also re-evaluated current type certification requirements for bird ingestion and how to address applicable NTSB recommendations related to this accident.
Based on the study, the FAA has published a final rule revision, for medium-size flocking birds, to Part 33 engine certification requirement § 33.76, at amendment 33-36, and revision B to Advisory Circular 33.76-1. The final rule requires that, to obtain certification of a turbofan engine, a manufacturer must show that the engine core can continue to operate after ingesting a medium-sized bird while operating at a lower fan speed associated with climb or landing.
The revised § 33.76 creates an additional bird ingestion test for turbofan engines. The new requirements are added to § 33.76, which covers engine testing for bird ingestion. This new test should ensure that engines can ingest the largest medium flocking bird (MFB), required by the existing rule, into the engine core at climb conditions. If the engine design is such that no bird material will be ingested into the engine core during the test at climb conditions, then the proposed rule would require a different test at approach conditions. The final rule and all comments received are publicly available on Regulations.gov, in docket FAA-2018-0568.
- December 14, 2018, EASA published "Certification Specification for Engines Amendment 5" in Executive Director Decision 2018/014/R, with similar requirements.
AIA Bird Ingestion Working Group Report
Crashworthiness and Ditching
- Transport Airplane Crashworthiness and Ditching ARAC Working Group, ARAC Working Group Report, September 20, 2018, made several recommendations, some of which are still under consideration at the FAA.
ARAC Transport Airplane Crashworthiness and Ditching Working Group Recommendation Report
- The EASA "Certification Specifications and Acceptable Means of Compliance for Large Aeroplanes (CS-25)," amendment 24, was published on January 13, 2020. CS-25.671(d). This amendment specifies that aircraft must be designed so that, if all engines fail at any time of the flight, a flare to a landing and a flare to a ditching can be achieved. In addition, CS-25.671, section 8, specifies that it should be possible to perform a flare to a safe landing and ditching attitude, in the most critical configuration, from a stabilized approach using the recommended approach speeds, pitch angles, and appropriate aircraft flight manual procedures, without requiring exceptional piloting skills or strengths.
Aircraft Structure
- Both FAA and EASA published airworthiness directives to require a design change that prevents the central vertical strut at frame 65 from penetrating the cabin floor (refer to Airworthiness Directives Issued section)
Checklists and Training
-
The FAA agreed with the NTSB that consideration of altitude should be included in the development of specific procedures for all-engines-out scenarios as a best practice. This expectation is now included in project-specific inflight engine restart guidance for new or major design change transport category airplane programs.
For new or major design change transport category airplane certification programs, the FAA expects project-specific inflight engine restart procedures (in the "Airplane Flight Manual") to address low-altitude restart events, including dual-engine failure that occurs at low altitude and during which the flight crew has limited time to address the failure before they must prepare for a forced landing.
-
On January 5, 2016, the FAA issued Notice N8900.339, "Actions Required to Identify and Correct Discrepancies Between Airplane Flight Manuals and Flight Deck Quick Reference Handbooks." The notice alerts principal operations inspectors (POIs) of discrepancies that exist between some AFMs and their associated QRHs and directs the POIs to review the QRHs for compliance with the FAA-approved abnormal/emergency procedures checklists found in the AFMs. The notice also defines POI actions in the event they discover discrepancies.
Although Notice N8900.339 expired on January 5, 2017, on March 15, 2016, FAA Order 8900.1, "Flight Standards Information Management System," volume 3, chapter 3, sections 5 and 12 was revised to include the guidance contained in the notice. These revisions will result in the discovery and resolution of the discrepancy found in the US Airways Flight 1549 checklists regarding turning off GPWS. The revision also covers additional forms of envelope protection, such as stick-shaker and stick-pusher training. The change to the Order informs POIs of the available manufacturer information on envelope protection and the need to evaluate operators' training programs. This evaluation must ensure that operators' training programs adequately discuss the effects of envelope protection during abnormal or emergency operations and incorporate these effects into flight training.
- The FAA issued Change 285 to Order 8900.1, effective August 28, 2013. This change added Paragraph 3-276 to Volume 3, Chapter 10, Section 1, "Operational Emphasis Items." The new paragraph includes information regarding reviewing and approving pilot training programs. It also clarifies that FAA inspectors must ensure that both ground and flight training must address the effects of envelope protection during normal, abnormal, and emergency operations.
- On February 3, 2017, the FAA published TSO C13g, "Life Preservers," which is consistent with the updated inflation requirements provided in Society of Automotive Engineers International Aerospace Standard 1354, "Individual Inflatable Life Preserver." The revised TSO includes improved performance and inspection criteria for life preservers that are donned multiple times a day, as well as contains requirements to prevent the inflation cylinder from backing out and to use a visual indicator to make inflation system inspection easier. The TSO includes new requirements to make it easier to understand the donning instructions on the life preserver, to operate the retention mechanism, and to open the life preserver packages. Further, the TSO includes testing that simulates the reduced dexterity that a person may experience during an emergency in a cold and wet environment.
Wildlife Deterrent Technology
- The FAA, with the USDA, continues to conduct research into innovative technologies for installation on aircraft to reduce the likelihood of a bird strike. As part of its comprehensive Wildlife Hazard Research and Development Program, the FAA is investigating pulsing aircraft lights and other strategies to deter birds from the airport environment, such as ultraviolet light emitting diodes (LEDs), pulsing LEDs, direct energy systems, and acoustic hailing devices. The FAA continues to investigate whether these technologies are useful for bird deterrent systems onboard aircraft. The FAA is also engaged in industry and government research and remains alert to promising developments in aircraft-installed technologies that demonstrate a reduction in the likelihood of bird strikes. Aircraft operators and manufacturers are currently able to procure and certify pulsating light systems and other deterrent systems that they may propose within the framework of the FAA's existing regulations and policies. The FAA continues working together with USDA to collect, track, and publish information about wildlife strikes, including bird strikes, affecting aviation and the national airspace.
USDA/FAA Wildlife Strikes to Civil Aircraft in the United States - 1990-2022
Passenger Briefing
- AC 121-24D - On March 5, 2019, FAA issued Advisory Circular (AC) 121-24D, Passenger Safety Information Briefing and Briefing Cards, which applies to 14 CFR Parts 121 and 135 operations. Appendix 4, Brace-for-Impact Positions, provides updated relevant information regarding required or recommended content for oral passenger safety information briefings, safety information briefing cards, and a variety of emergency procedures. AC 121-24D also includes updated information about oral passenger safety briefings and safety information briefing cards, including the use of life lines.
The European Aviation Safety Agency (EASA), the primary certification authority, issued Airworthiness Directive (AD) 2016-0212 on October 25, 2016, requiring modification of the fuselage structure at frame 65, in accordance with the applicable Airbus service bulletins. The FAA issued AD 2017-25-06, Airbus Airplanes, on December 11, 2017. This required design change prevents the central vertical strut at frame 65 from penetrating the cabin floor, which could cause injury to occupants and delay in emergency evacuations.
Airplane Life Cycle
- Operational
Accident Threats
- Flight Deck Layout / Avionics Confusion
Groupings
- N/A
Accident Common Themes
- Flawed Assumptions
Flawed Assumptions
Dual-engine loss would most likely occur at higher cruise altitudes, and likelihood of low-altitude, dual-engine loss was sufficiently low.
- This dual-engine loss accident occurred at approximately 2,800 feet altitude.
Training for dual-engine loss at high altitude was sufficient for most occurrences.
- Training for dual-engine loss at high altitude, with one engine recovered, was inadequate to prepare pilots for a dual-engine loss at 2,800 feet, with no possibility to recover an engine.
Checklist for dual-engine loss at high altitude would be sufficient for most occurrences and pilots would have time to get thru the checklist before the decision to ditch.
- Checklist for dual-engine loss at high altitude, being lengthy and complex, was impractical for a dual-engine loss at 2,800 feet, and subsequent ditching.
Large birds would not enter both engine cores in the same strike.
- Multiple large birds (migratory Canada geese) entered the engine cores of both engines on Flight 1549, and required testing did not account for this situation.
See Precursors.
Technical Related Lessons
The pilot's awareness of aircraft performance capability, if all thrust is lost at low altitude, is critical when deciding how to respond in an emergency. (Threat Category: Flight Deck Layout / Avionics Confusion)
- Evolving innovations in safety technology incorporated into aircraft design may reduce the risk of catastrophic outcomes and help pilots determine parameters, such as engine failure status, landing sites within an aircraft's range, and other critical information.
- New technology exists that can aid the pilot with the decision-making process by showing the aircraft’s flight path relative to approaching terrain and performance parameters on aircraft displays. This technology could allow pilots to quickly narrow their options, reduce workload, save time, and increase the chance of a successful outcome.
- The US Airways crew was task saturated during this low altitude and subsequent short-timeframe event. They were dealing with declaring an emergency, trying to run emergency checklists, and determining if they could return to LaGuardia or land somewhere else. The crew had only skill, experience, and training to help them decide if they could fly unpowered back to LaGuardia or any alternative airport.
Common Theme Related Lessons
Training and checklists can be vital to successful outcomes during emergencies. Each must be designed to address the most critical flight scenarios, and do so with expediency, as appropriate, to handle the associated emergency. (Common Theme: Flawed Assumption)
- The aircraft manufacturer assessed that the largest percentage of flight time for most flights is at cruise altitude. Since, based only on time-in-phase, the highest likelihood of dual-engine loss would occur during cruise, the manufacturer assumed that training pilots for only these conditions would sufficiently prepare them for less likely, yet more critical, takeoff or low-altitude, dual-engine loss.
- The pilots were forced to assess the situation, make decisions, and take appropriate action without the benefit of training specific to their scenario, thus increasing workload and stress.
- Emergency and non-normal checklists related to this accident were designed toward two scenarios. The first scenario is a high-altitude, dual-engine loss of thrust, where time to respond to the emergency and accomplish any associated procedures is plentiful. The second scenario is designed with a single-engine (rather than dual-engine) loss of thrust at takeoff or low altitude, where the remaining operating engine continues to provide sufficient thrust, and time is not a constraint. The more problematic, low-altitude, dual-engine loss of thrust did not factor into any non-normal checklist creation.
- Brevity in critical checklists and associated operational training can reduce crew workload and stress during actual emergencies. The dual-engine failure checklist for Flight 1549 did not allow completion of most steps due to length and complexity. This forced the flight crew to assess the situation, make decisions, and take appropriate action without benefit of a checklist for their scenario, thus increasing workload and stress.